Genetics of Endocrine and Neuroendocrine Neoplasias (PDQ®)–Health Professional Version
Executive Summary
This executive summary reviews the topics covered in this PDQ summary on the genetics of endocrine and neuroendocrine neoplasias, with hyperlinks to detailed sections below that describe the evidence on each topic.
- Inheritance and Risk
Several hereditary syndromes involve the endocrine or neuroendocrine glands. Multiple endocrine neoplasia type 1 (MEN1), multiple endocrine neoplasia type 2 (MEN2), multiple endocrine neoplasia type 4 (MEN4), familial pheochromocytoma (PHEO) and paraganglioma (PGL) syndrome (FPPL), Carney-Stratakis syndrome (CSS), and familial nonmedullary thyroid cancer (FNMTC) are discussed in this summary. Autosomal dominant pathogenic variants cause most of these syndromes. PHEOs and PGLs may also be found in individuals with von Hippel-Lindau disease. For more information, see von Hippel-Lindau Disease.
- Associated Genes and Syndromes
MEN1, which is primarily associated with the development of parathyroid tumors and primary hyperparathyroidism, duodenopancreatic neuroendocrine tumors (NETs), and pituitary tumors, is caused by germline pathogenic variants in the MEN1 gene. The primary endocrine features of MEN2, which is subdivided into MEN2A and MEN2B, include medullary thyroid cancer (MTC); its precursor, C-cell hyperplasia; PHEO; and parathyroid adenomas and/or hyperplasia. MEN2 is caused by germline pathogenic variants in the RET gene. MEN4 is a rare syndrome with clinical features that overlap with the other MEN syndromes; the most common features are primary hyperparathyroidism and pituitary adenomas. MEN4 is caused by germline pathogenic variants in the CDKN1B gene. Both FPPL and CSS are caused by germline pathogenic variants in the SDH genes. PHEOs and PGLs commonly occur sporadically as well, although up to 33% of apparently sporadic PHEOs in individuals with no known family history and up to 40% of apparently sporadic PGLs have a recognizable germline pathogenic variant in one of the known PGL/PHEO susceptibility genes. Multifocal, locally aggressive gastrointestinal stromal tumors (GISTs) are also found in individuals with CSS. FNMTC is a polygenic disease with no single locus responsible for the majority of cases or easily identifiable phenotype and is likely modified by multiple low-penetrance alleles and environmental factors.
- Clinical Management
Regular surveillance is a mainstay in individuals found to have or be at risk of carrying a pathogenic variant in MEN1, RET, CDKN1B, or one of the SDH genes. Surveillance recommendations include regular screening for both endocrine and nonendocrine manifestations of disease.
Surgical management of pituitary and parathyroid tumors in MEN1 is based on disease presentation and management of symptoms of the organ. Surgical management of duodenopancreatic NETs of MEN1 is more specific to preventing disease progression.
The decision to operate on PHEOs in MEN2 is based on hormonal hypersecretion and symptomatology. Treatment of MTC consists of surgical removal of the entire thyroid gland, including the posterior capsule, and central lymph node dissection. In addition, risk-reducing thyroidectomy has been shown to reduce the subsequent incidence of persistent or recurrent disease in MEN2 patients who had thyroidectomy earlier in life. The timing of risk-reducing thyroidectomy is guided by the risks associated with specific RET variants, although basal calcitonin levels may be used to determine the optimal timing of the procedure. MEN2-related parathyroid disease may also be treated surgically or with medical therapy in high-risk surgical patients.
Parathyroid and pituitary tumors associated with MEN4 are also managed surgically, in accordance with treatment for other familial syndromes such as MEN1.
FPPL-associated PHEOs and PGLs are also treated surgically. Preoperative management aimed at preventing catecholamine-induced complications of the surgery is common.
The mainstay of treatment for CSS-associated GISTs and PGLs is complete surgical resection of the tumor. The timing of the operation correlates with the presentation of the tumor.
Thyroid cancers associated with FNMTC are also managed surgically, commonly with a total thyroidectomy. Patients who undergo a total thyroidectomy must receive lifelong thyroid hormone replacement therapy.
Introduction
There are several hereditary syndromes that involve endocrine or neuroendocrine glands, such as multiple endocrine neoplasia type 1 (MEN1), multiple endocrine neoplasia type 2 (MEN2), multiple endocrine neoplasia type 4 (MEN4), pheochromocytoma (PHEO), paraganglioma (PGL), Li-Fraumeni syndrome, familial adenomatous polyposis, and von Hippel-Lindau disease. This summary currently focuses on MEN1, MEN2, MEN4, familial PHEO and PGL syndrome, Carney-Stratakis (CSS) syndrome, and familial nonmedullary thyroid cancer (FNMTC). Li-Fraumeni syndrome, familial adenomatous polyposis, Cowden syndrome, and von Hippel-Lindau disease are discussed in the following PDQ summaries: Genetics of Breast and Gynecologic Cancers, Genetics of Colorectal Cancer, and von Hippel-Lindau Disease.
The term multiple endocrine neoplasia is used to describe a group of heritable tumors in endocrine tissues, which can be benign or malignant. Multiple endocrine neoplasias are typically classified into two main categories: MEN1 (also known as Wermer syndrome) and MEN2. Historically, MEN2 was further divided into three subtypes based on the presence or absence of certain endocrine tumors in an individual or family: MEN2A, familial medullary thyroid cancer (FMTC), and MEN2B (which is sometimes referred to as MEN3). FMTC is now considered a subtype of MEN2A.[1] MEN4 was described as a novel syndrome in humans in 2011. Major characteristics of MEN4 include primary hyperparathyroidism and pituitary adenomas. MEN syndrome–associated tumors usually manifest as the overproduction of hormones, tumor growth, or both. For more information, see the MEN1, MEN2, and MEN4 sections.
PGLs and PHEOs are rare tumors arising from chromaffin cells, which have the ability to synthesize, store, and secrete catecholamines and neuropeptides.[2] Either tumor may occur sporadically, as a manifestation of a hereditary syndrome, or as the sole tumor in familial PGL and PHEO syndrome. For more information, see the Familial PHEO and PGL Syndrome section.
Affected individuals with Carney-Stratakis syndrome (CSS) have multifocal, locally aggressive gastrointestinal stromal tumors and multiple neck, intrathoracic, and intra-abdominal PGLs at relatively young ages.[3-5] CSS is distinct from similarly named syndromes, Carney Complex and Carney Triad. For more information, see the CSS section.
Familial nonmedullary thyroid cancer (FNMTC) is thought to account for 5% to 10% of all differentiated thyroid cancer cases.[6-8] With the exception of a few rare genetic syndromes that include nonmedullary thyroid cancer as a minor component, most FNMTC is nonsyndromic, and the underlying genetic predisposition is unclear. For more information, see the FNMTC section.
References
- Wells SA, Asa SL, Dralle H, et al.: Revised American Thyroid Association guidelines for the management of medullary thyroid carcinoma. Thyroid 25 (6): 567-610, 2015. [PUBMED Abstract]
- Inherited tumour syndromes. In: Lloyd RV, Osamura RY, Klöppel G, et al.: WHO Classification of Tumours of Endocrine Organs. 4th ed. International Agency for Research on Cancer, 2017, pp. 262–66.
- Carney JA, Stratakis CA: Familial paraganglioma and gastric stromal sarcoma: a new syndrome distinct from the Carney triad. Am J Med Genet 108 (2): 132-9, 2002. [PUBMED Abstract]
- McWhinney SR, Pasini B, Stratakis CA, et al.: Familial gastrointestinal stromal tumors and germ-line mutations. N Engl J Med 357 (10): 1054-6, 2007. [PUBMED Abstract]
- Pasini B, McWhinney SR, Bei T, et al.: Clinical and molecular genetics of patients with the Carney-Stratakis syndrome and germline mutations of the genes coding for the succinate dehydrogenase subunits SDHB, SDHC, and SDHD. Eur J Hum Genet 16 (1): 79-88, 2008. [PUBMED Abstract]
- Stoffer SS, Van Dyke DL, Bach JV, et al.: Familial papillary carcinoma of the thyroid. Am J Med Genet 25 (4): 775-82, 1986. [PUBMED Abstract]
- Mazeh H, Sippel RS: Familial nonmedullary thyroid carcinoma. Thyroid 23 (9): 1049-56, 2013. [PUBMED Abstract]
- Lupoli G, Vitale G, Caraglia M, et al.: Familial papillary thyroid microcarcinoma: a new clinical entity. Lancet 353 (9153): 637-9, 1999. [PUBMED Abstract]
Multiple Endocrine Neoplasia Type 1
Clinical Description
Multiple endocrine neoplasia type 1 (MEN1) is an autosomal dominant syndrome, with an estimated prevalence of about 1 in 30,000 individuals.[1] The major endocrine features of MEN1 include the following:
A clinical diagnosis of MEN1 may be made when an individual has two of the three major endocrine tumors listed above, especially if he/she was diagnosed with these tumors at a young age. Alternatively, familial MEN1 may be defined as having at least one MEN1 case in the family plus at least one first-degree relative (FDR) with one of these three tumors, or two FDRs with a germline pathogenic variant.[2-5]
Initial clinical presentation of symptoms typically occurs between the ages of 20 and 30 years. However, in many cases, an MEN1 diagnosis may not be confirmed for many years after initial symptoms occur. The age-related penetrance of MEN1 is 45% to 73% by age 30 years, 82% by age 50 years, and 96% by age 70 years.[2,5-7] To date, there are no well-established genotype-phenotype correlations to guide clinical management of patients with MEN1.
Parathyroid Tumors and PHPT
The most common features and often the first presenting signs of MEN1 are parathyroid tumors, which result in PHPT. These tumors occur in 80% to 100% of patients by age 50 years.[8,9] MEN1-associated parathyroid tumors are typically multiglandular and hyperplastic. This differs from sporadic parathyroid tumors, which often present with a solitary adenoma.[10] The mean age of PHPT onset is 20 to 25 years in individuals with MEN1. In contrast, PHPT onset occurs in the general population at age 50 to 59 years. When MEN1 presents in childhood, the most common presenting feature is multi-gland hyperparathyroidism.[11] Parathyroid carcinoma in MEN1 is rare but has been described.[12-14]
Individuals with MEN1-associated PHPT will have elevated parathyroid hormone (PTH) and calcium levels in the blood. The clinical manifestations of PHPT are mainly the result of hypercalcemia. Mild hypercalcemia may go undetected and have few or no symptoms. More severe hypercalcemia can result in the following:
- Constipation.
- Nausea and vomiting.
- Dehydration.
- Decreased appetite and abdominal pain.
- Anorexia.
- Diuresis.
- Kidney stones.
- Increased bone resorption with resultant increased risk of bone fracture.
- Lethargy.
- Depression.
- Confusion.
- Hypertension.
- Shortened QT interval.
Since MEN1-associated hypercalcemia is directly related to the presence of parathyroid tumors, surgical removal of these tumors may normalize calcium and PTH levels. This can help relieve an individual's symptoms. However, there have been high recurrence rates of parathyroid tumors after surgery in some series.[15-17] For more information, see the Interventions section.
Duodenopancreatic NETs
Duodenopancreatic NETs are the second most common endocrine manifestation in MEN1, occurring in 30% to 80% of patients by age 40 years.[2,8] A study has shown that the incidence may be as great as twofold higher in young patients (aged 20–40 y) with pathogenic variants in exon 2 of MEN1. These individuals are also more likely to have more aggressive disease and distant metastases.[18] Furthermore, duodenopancreatic NETs are associated with early mortality even after surgical resection.[19]
Duodenopancreatic NETs seen in MEN1 include the following:
- Gastrinomas.
- Nonfunctioning NETs.
- Insulinomas.
- Vasoactive intestinal peptide tumors (VIPomas).
- Glucagonomas.
- Somatostatinomas.
Tumor type | Estimated Penetrance | Symptoms |
---|---|---|
MEN1 = multiple endocrine neoplasia type 1. | ||
Gastrinoma | ≤70% [8,20] | Peptic ulcer disease and esophagitis |
Diarrhea | ||
Abdominal pain | ||
Weight loss | ||
Nonfunctioning | 20%–55% [8,21] | Local compressive symptoms: abdominal pain, jaundice, anorexia, weight loss |
Insulinoma | 10% [8] | Whipple’s triad: symptomatic hypoglycemia reversed by glucose administration with associated elevation of insulin, C-peptide, and proinsulin levels |
Vasoactive intestinal peptide | 1% [8,22] | Watery diarrhea |
Hypokalemia | ||
Achlorhydria | ||
Glucagonoma | 1% [8,22] | Diabetes mellitus |
Diarrhea | ||
Depression | ||
Necrolytic migratory erythema | ||
Thromboembolic disease | ||
Somatostatinoma | <1% [22] | Diabetes mellitus |
Diarrhea/steatorrhea | ||
Gallbladder disease | ||
Hypochlorhydria | ||
Weight loss |
Gastrinomas represent 50% of the gastrointestinal NETs in MEN1 and are the major cause of morbidity and mortality in MEN1 patients.[2,15] Gastrinomas are usually multicentric, with small (<0.5 cm) foci throughout the duodenum.[23] Most result in peptic ulcer disease (Zollinger-Ellison syndrome), and half are malignant at the time of diagnosis.[5,15,23,24]
Originally, nonfunctioning duodenopancreatic NETs were thought to be uncommon in individuals with MEN1. However, recognition of these tumors has increased with advanced genetic testing and improved imaging techniques. For example, a prospective study showed that MEN1 pathogenic variant carriers had a nonfunctioning duodenopancreatic NET frequency of 55% by age 39 years when they underwent endoscopic ultrasonography of the pancreas.[21,25] These tumors can be metastatic. One study of 108 MEN1 pathogenic variant carriers with nonfunctioning duodenopancreatic NETs showed a positive correlation between tumor size, rate of metastasis, and death. Individuals with tumors larger than 2 cm had significantly higher rates of metastasis than those with tumors smaller than 2 cm.[26] For more information, see the Molecular Genetics of MEN1 section.
Pituitary Tumors
Approximately 15% to 50% of MEN1 patients will develop a pituitary tumor.[2,8] Two-thirds are microadenomas (<1.0 cm in diameter), and the majority are prolactin-secreting.[27] Other pituitary tumors can include somatotropinomas and corticotropinomas, or they may be nonfunctioning.
Tumor type | Estimated Penetrance | Symptoms |
---|---|---|
MEN1 = multiple endocrine neoplasia type 1. | ||
Prolactinoma | 20% [8] | Galactorrhea |
Amenorrhea/infertility | ||
Hypogonadism | ||
Somatotropinoma | 10% [8] | Coarse facial features |
Soft tissue overgrowth: enlargement of hands/feet | ||
Hyperhidrosis | ||
Corticotropinoma | <5% [8] | Weight gain |
Hypertension | ||
Flushing | ||
Easy bruising/bleeding | ||
Hyperglycemia |
Other MEN1-Associated Tumors
Other manifestations of MEN1 include carcinoids of the foregut (5%–10% of MEN1 patients). These are typically bronchial or thymic and are sometimes gastric. Skin lesions are also common and can include facial angiofibromas (up to 80% of MEN1 patients) and collagenomas (~75% of MEN1 patients).[28] Lipomas (~30% of MEN1 patients) and adrenal cortical lesions (up to 50% of MEN1 patients),[29] including cortical adenomas, diffuse or nodular hyperplasia, or rarely, carcinoma are also common.[30-32] The following manifestations have also been reported:[33-35]
- Thyroid adenoma.
- Pheochromocytoma.
- Spinal ependymoma.
- Meningioma.
- Leiomyoma (e.g., esophageal, lung, and uterine).
Making the Diagnosis of MEN1
MEN1 is often difficult to diagnose in the absence of a significant family history or a positive genetic test for a pathogenic variant in the MEN1 gene. One study of 560 individuals with MEN1 showed a significant delay between the time of the first presenting symptom and the diagnosis of MEN1.[36] This time lapse is likely because some presenting symptoms of MEN1-associated tumors, such as amenorrhea, peptic ulcers, hypoglycemia, and nephrolithiasis, are not specific to MEN1.
Furthermore, identification of an MEN1-associated tumor is not sufficient to make the clinical diagnosis of MEN1 and may not trigger a referral to an endocrinologist. The median time between the first presenting symptom and diagnosis of MEN1 ranges from 7.6 years to 12 years.[6,31] Genetic testing alleviates some of this delay. Several studies have shown statistically significant differences in the age at MEN1 diagnosis between probands and their family members. In one study, clinically symptomatic probands were diagnosed with MEN1 at a mean age of 47.5 years (standard deviation [SD] +/- 13.5 y), while family members were diagnosed at a mean age of 38.5 years (SD +/- 15.4 y; P < .001).[36] In another study of 154 individuals with MEN1, probands were diagnosed at a mean age of 39.5 years (range: 18–74 y), compared with a mean age of 27 years (range: 14–56 y; P < .05) in family members diagnosed by predictive genetic testing.[37] Nonetheless, the lag time between the diagnosis of MEN1 in an index case and the diagnosis of MEN1 in family members can be significant, leading to increased morbidity and mortality.[38] This was demonstrated in a Dutch MEN1 Study Group analysis, which showed that 10% to 38% of non-index cases already had an MEN1-related manifestation at diagnosis; 4% of these individuals died of an MEN1-related cause that developed during or before the lag time. In family members, the majority of the morbidity related to lag time was due to metastatic duodenopancreatic NETs, pituitary macroadenomas, and multiple MEN1 manifestations.[38] Early intervention is particularly critical as it relates to mortality from duodenopancreatic NETs. A study showed that for every year older at time of surgery, the odds of metastasis increased by 6%.[19] These findings underscore the importance of increased awareness of the signs and symptoms of MEN1-related tumors and the constellation of findings necessary to suspect the diagnosis. It also highlights the importance of genetic counseling and testing and communication among family members once a diagnosis of MEN1 is made.[39,40] Figure 1 illustrates some of the challenges in identifying MEN1 in a family.
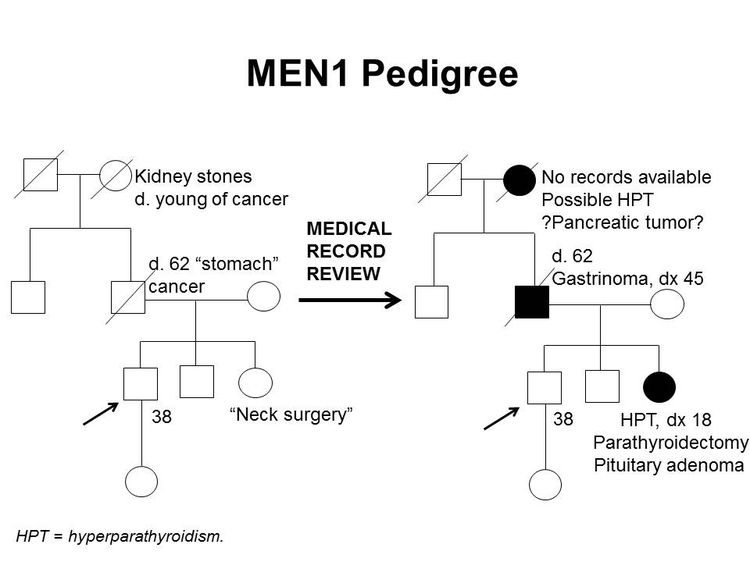
Since many of the tumors in MEN1 are underdiagnosed or misdiagnosed, identifying an MEN1 gene pathogenic variant in the proband early in the disease process can allow for early detection and treatment of tumors and earlier identification of at-risk family members. Many studies have been performed to determine the prevalence of MEN1 gene pathogenic variants among patients with apparently sporadic MEN1-related tumors.[8] For example, approximately one-third of patients with Zollinger-Ellison syndrome will carry an MEN1 pathogenic variant.[41,42] In individuals with apparently isolated PHPT or pituitary adenomas, the pathogenic variant prevalence is lower, on the order of 2% to 5%,[27,43,44] but the prevalence is higher in individuals diagnosed with these tumors before age 30 years. Some authors suggest referral for genetics consultation and/or genetic testing for pathogenic variants in MEN1 if one of the following conditions is present:[8,45-47]
- Gastrinoma at any age in the individual or an FDR.
- Multifocal duodenopancreatic NETs at any age.
- PHPT before age 30 or 40 years.
- Multiglandular parathyroid adenomas/hyperplasia or recurrent PHPT.
- Presence of one of the three main MEN1 tumors plus one of the less common tumors/findings.
- Presence of two or more features (e.g., adrenal adenomas and carcinoid tumor).
- Combination of at least two of the following in one individual: parathyroid adenoma; thymic, bronchial, or foregut carcinoid tumor; duodenopancreatic NET; pituitary tumor; adrenal tumor.
- Parathyroid adenoma and a family history of hyperparathyroidism, pituitary adenoma, duodenopancreatic NET, or foregut carcinoid tumor.
- Multiple primary duodenopancreatic NETs in the same person.
Molecular Genetics of MEN1
The MEN1 gene is located on chromosome 11q13 and encodes the protein menin.[3,48,49] Over 1,300 pathogenic variants have been identified in the MEN1 gene to date, and these are scattered across the entire coding region.[50,51] Most (~65%) of these are nonsense or frameshift variants. The remainder are missense variants (20%) (which lead to the expression of an altered protein), splice-site variants (9%), or partial- or whole-gene deletions (1%–4%).[52] In MEN1, phenotypic variation is common within a family and between unrelated individuals. Data suggested that anticipation may also occur in families with MEN1 pathogenic variants.[8,53-55] One large study demonstrated that pituitary, adrenal, and thymic NETs had the highest rates of heritability.[56]
Genetic Testing and Differential Diagnosis for MEN1
Genetic testing for MEN1 pathogenic variants is recommended for individuals meeting clinical diagnostic criteria and may be considered in individuals with less common MEN1-associated tumors. For more information, see the Making the diagnosis of MEN1 section. For individuals meeting diagnostic criteria, the pathogenic variant detection rate is approximately 75% to 90%.[53,57] Still, germline pathogenic variant yield ranged from 16% to 38% for apparently sporadic cases of parathyroid (15.8%), pancreatic islet (25.0%), or pituitary (37.5%) tumors. Genetic testing may be warranted in these individuals because a diagnosis of MEN1 would prompt screening for other MEN1-related tumors.[58] Laboratories that offer MEN1 testing primarily use DNA sequencing. Several laboratories offer additional analyses for MEN1 partial- or whole-gene deletion and/or duplication. However, these types of variants are rare. Deletion/duplication testing is often reserved for individuals who have very high clinical suspicions for MEN1 but a detectable pathogenic variant was not found by direct DNA sequencing. Evolving studies continue to reveal causative pathogenic variants in MEN1.[52]
A multigene panel that includes MEN1 and other genes associated with an increased risk of endocrine tumors may also be used. Such genetic testing can be used to distinguish between MEN1 and other forms of hereditary hyperparathyroidism, such as familial isolated hyperparathyroidism (FIHP), hyperparathyroidism–jaw tumor syndrome (HPT-JT), and familial hypocalciuric hypercalcemia (FHH). [Note: The hyperparathyroidism in FHH is not primary hyperparathyroidism, which is seen in MEN1, HPT-JT and FIHP.] HPT-JT, which is caused by germline pathogenic variants in the CDC73 gene, is associated with PHPT, ossifying lesions of the maxilla and mandible, and renal lesions, usually bilateral renal cysts, hamartomas, and in some cases, Wilms tumor.[59,60] Unlike MEN1, HPT-JT is associated with an increased risk of parathyroid carcinoma.[61] FIHP, as its name suggests, is characterized by isolated PHPT with no additional endocrine features; in some families, FIHP is the initial diagnosis of what later develops into MEN1, HPT-JT, or FHH.[62-64] Approximately 20% of families with a clinical diagnosis of FIHP carry germline MEN1 pathogenic variants.[63,65,66] Pathogenic variants in the calcium-sensing receptor (CASR) gene cause FHH, which can closely mimic the hyperparathyroidism seen in MEN1.
Genetic diagnosis will help guide management for patients with early-onset hyperparathyroidism. This is especially crucial, since many of the above conditions have different management guidelines that correspond with their features. For example, distinguishing between MEN1 and FHH can be critical for a patient's disease management. Removing the parathyroid glands in FHH does not correct the hyperparathyroidism that is seen in patients with MEN1. This could result in an unnecessary surgery that would not relieve the patient's symptoms. In addition, HPT-JT is unique because it increases parathyroid carcinoma risk. Hence, individuals with this syndrome have different management guidelines than individuals with other forms of hereditary hyperparathyroidism.[67,68] For more information on MEN1 clinical features and other forms of hyperparathyroidism, see Table 3.
Condition | Gene(s) | Major Clinical Features |
---|---|---|
FHH = familial hypocalciuric hypercalcemia; FIHP = familial isolated hyperparathyroidism; HPT-JT = hyperparathyroidism–jaw tumor syndrome; MEN1 = multiple endocrine neoplasia type 1 (gene is italicized); NETs = neuroendocrine tumors; PHPT = primary hyperparathyroidism. | ||
MEN1 | MEN1 | PHPT, pituitary adenomas, duodenopancreatic NETs [8,10,69] |
FIHP | MEN1, CDC73 | PHPT [62-66] |
HPT-JT | CDC73 | PHPT; osteomas of maxilla and mandible; renal cysts or hamartomas; and rarely, Wilms tumor and parathyroid carcinoma [59-61] |
FHH | CASR (type 1), GNA11 (type 2), AP2S1 (type 3) | Hyperparathyroidism (not primary) [67,70-72] |
Surveillance
Screening and surveillance for MEN1 may include a combination of biochemical tests and imaging techniques.
Traditionally, magnetic resonance imaging (MRI) was used for surveillance and staging. However, ongoing studies have evaluated the role of MRI in functional imaging, including gallium Ga 68-DOTATATE (68Ga-DOTATATE) positron emission tomography (PET)–computed tomography (CT) scanning. A multicenter retrospective study examined 108 MEN1 patients undergoing PET-CT for screening, staging, restaging, or targeted radiotherapy selection. This study demonstrated that PET-CT has the potential to increase diagnostic sensitivity when searching for MEN1-associated NETs.[73] In 51% of cases, PET-CT provided superior lesion detection when compared with conventional imaging techniques. However, the retrospective nature of the study makes it impossible to discern how much selection bias may have impacted the study's findings. Consequently, PET-CT's exact role in detecting metastasis remains unclear. PET-CT's potentially improved diagnostic sensitivity must also be weighed against its increased levels of radiation exposure, which are higher than that of other imaging modalities. Radiation exposure is particularly relevant for MEN1 patients, who are prone to developing aggressive malignancies. The issue is even more nuanced in young patients who require lifelong screening to detect aggressive MEN1-associated malignancies early.
A study analyzed thoracic screening techniques in 50 patients with MEN1. It found that when patients with MEN1 underwent functional imaging with fluorine F 18-fludeoxyglucose (18F-FDG) PET-CT screening, they had a similar number of lung nodules as individuals in the general population. However, when lesions in MEN1 patients were FDG-avid, they were more likely to progress during the follow-up period. Therefore, further observation and follow up of FDG-avid lesions may be warranted in patients with MEN1.[74] While lung-specific imaging is not routinely performed in MEN1 patients, the lungs are visualized during MRI surveillance of thymic lesions. When pulmonary lesions are identified, it is important to recognize that lung NETs may grow slowly and may have a good prognosis. Referral to a multidisciplinary team may be beneficial for selective resection of lung NETs.[75]
Recommendations for MEN1 surveillance are summarized in Table 4.[4,8]
Biochemical Test or Procedure | Condition Screened For | Age Screening Initiated (y) | Frequency |
---|---|---|---|
CT = computed tomography; MEN1 = multiple endocrine neoplasia type 1; MRI = magnetic resonance imaging; NETs = neuroendocrine tumors; PHPT = primary hyperparathyroidism; PTH = parathyroid hormone. | |||
aAdapted from Brandi et al.[4] and Thakker et al.[8] | |||
bThe recommendations for abdominal imaging differ between two published guidelines for the diagnosis and management of MEN1.[4,8] There is weak evidence at this time to support annual imaging before age 10 years. Imaging before age 10 years does identify disease in a high proportion of patients, but it is not clear whether this impacts prognosis.[21,76] | |||
cThe age to initiate screening and the screening frequency for pituitary tumors may be debatable because the clinical significance of small, nonfunctional tumors is unclear;[77] further study may be warranted. | |||
dAdapted from Niederle et al.[5] | |||
eAdapted from Shirali et al.[78] The 2012 guidelines recommend chest MRI every 1-2 years.[8] | |||
Serum prolactin and/or insulin-like growth factor 1 | Pituitary tumors | 5 | Every 1 y |
Fasting total serum calcium and/or ionized calcium and PTH | Parathyroid tumors and PHPT | 8 | Every 1 y |
Fasting serum gastrin | Duodenopancreatic gastrinoma | 20 | Every 1 y |
Chromogranin A, pancreatic polypeptide, glucagon, and vasointestinal polypeptided | Duodenopancreatic NETs | 10–16 | Up to every 3 years (consider every 3 years if asymptomatic; consider shorter screening intervals depending on the clinical scenario) |
Fasting glucose and insulin | Insulinoma | 5 | Every 1 y |
Brain MRIc | Pituitary tumors | 5 | Every 3–5 y based on biochemical results |
Chest MRIe | Thymic and bronchial NETs | <20 | About every 3 years. Consider more frequent screening for men, smokers, or individuals with a positive family history. Baseline chest MRI is done prior to parathyroidectomy |
Abdominal CT or MRIb [4] | Duodenopancreatic NETs | 20 | Every 3–5 y based on biochemical results |
Abdominal CT, MRI, or endoscopic ultrasonographyb [8] | Duodenopancreatic NETs | <10 | Every 1 y |
Interventions
Surgical management of MEN1 is complex and controversial, given the multifocal and multiglandular nature of the disease. Patients with MEN1 have a high risk of tumor recurrence, even after surgery. Additionally, these patients may have an increased risk of developing venous thromboembolisms.[79] Clinicians should be aware of this risk, particularly in the perioperative period. It is critical to establish an MEN1 diagnosis before making surgical decisions, in order to prevent unnecessary and/or inappropriate surgeries. Furthermore, it is recommended that individuals with MEN1 use a surgeon who has experience treating this disease.
Treatment for parathyroid tumors
Once evidence of parathyroid disease is established biochemically, surgical removal of the hyperfunctional parathyroid tissue is recommended to achieve eucalcemia and euparathyroidism. However, the timing and the amount of parathyroid and thymus gland tissue that is removed during surgery remains controversial.[40] For patients with primary hyperparathyroidism who are at risk for MEN1, preoperative detection of a pathogenic variant helps guide the extent of their initial operations, can increase the likelihood of successful initial surgeries, and lower the likelihood of recurrent disease.[68] Furthermore, knowledge of an MEN1 pathogenic variant can help guide surgical decision making and avoid the use of a single-gland surgical approach. Studies have shown that concomitant bilateral cervical thymectomy decreases the rate of parathyroid tumor recurrence and suggests that the thymus can be removed during the patient's initial operation.[80]
Some groups reserve surgical intervention for symptomatic patients, with continued annual biochemical screening for those without objective signs of disease. Subtotal parathyroidectomy (removal of 3–3.5 glands) is commonly suggested as the initial surgical treatment when a provider decides to proceed with surgery.[68] If 3.5 or more glands are removed during surgery, the rate of persistent disease is only 5% to 6%. Removing fewer than 3.5 glands decreases the durability of eucalcemia. Studies suggest that preoperative imaging (to determine which glands are hyperfunctional) is not reliable enough to justify unilateral exploration, with 86% of patients having enlarged contralateral parathyroid glands.[81] Fifty percent of the patients who had imaging to direct resection had the largest parathyroid gland identified intraoperatively on the contralateral side of greatest uptake.[81] Insufficient resection fails to accomplish the desired eucalcemia.[15-17,68]
Total parathyroidectomy with autotransplantation of parathyroid tissue to a distant site, such as the forearm, is a less commonly recommended option. Likelihood of cancer recurrence is lowered with total parathyroidectomy. However, this procedure also renders the patient aparathyroid for a period of time while the autotransplanted tissue becomes functional. This can cause a permanent PTH deficiency (no detectable PTH in the body).[80,82] Benefits of this approach include the following: 1) it is easier remove/debulk recurrent disease from the forearm than it is to remove/debulk recurrent disease from the neck, and 2) differential lateralization with arm blood draws. Hypocalcemia management involves taking numerous oral medications, including calcitrol and calcium replacement, and these daily requirements can be a major burden for patients. Recovery was less likely for patients who were aparathyroid for 6 months after total parathyroidectomy.[83] In select high-risk patients, like in those who had reoperative parathyroidectomy or cervical surgery, cryopreservation can be beneficial, but it is recommended that providers use this method sparingly.
Treatment for duodenopancreatic NETs
The timing and extent of surgery for duodenopancreatic NETs are controversial and depend on many factors, including severity of symptoms, extent of disease, functional component, location and necessity of simple enucleation, subtotal or total pancreatectomy, and pancreaticoduodenectomy (Whipple procedure). Surgical enucleation has been associated with higher recurrence compared with distal pancreatectomy, and a decreased rate of endocrine insufficiency compared with a Whipple procedure.[84] Tumor size has been suggested to advocate for surgical resection on the basis of the increased propensity for risk of metastases or recurrence with increased tumor diameter.[5,85,86] Unfortunately, there is no specific tumor marker or combination of tumor markers that are predictive of disease-specific mortality.[87] Long-acting somatostatin analogs may have a role in early-stage MEN1 duodenopancreatic NETs.[88] Initial study results of pharmacological therapy suggest that the treatment is safe and that long-term suppression of tumor and hormonal activity can be seen in up to 10% of patients and stability of hormone hyperfunction in 80% of patients.[88] The primary goal of surgery is to improve long-term survival by reducing symptoms associated with hormone excess and lowering the risk of distant metastasis.[24] Surgery is commonly performed for most functional tumors and for nonfunctioning NETs when the tumor exceeds 2 to 3 cm because the likelihood of distant metastases is high.[86,89-91] Structural imaging modalities alone are suboptimal for predicting the malignant potential of duodenopancreatic NETs. However, a study found that screening MEN1 patients with 18F-FDG PET-CT identified those NETs with an increased malignant potential; the FDG avidity correlated with a Ki-67 index.[92] Tumor size does seem to influence patient survival, with patients with smaller tumors having increased survival after resection.[93] While more-extensive surgical approaches (e.g., pancreatoduodenectomy) have been associated with higher cure rates and improved overall survival,[94-96] they also have higher rates of postoperative complications and long-term morbidity.[97] Therefore, the risks and benefits should be carefully considered, and surgical decisions should be made on a case-by-case basis. With regard to open or laparoscopic approaches, in selected patients, pancreatic laparoscopic surgery appears to be safe and associated with a shorter length of stay and fewer complications.[98]
Individuals with MEN1 who are diagnosed with NETs often have multiple tumors of various types throughout the pancreas and duodenum, some of which can be identified using magnetic resonance imaging or computed tomography (CT). Combining functional tracer accumulation with anatomic imaging improves tumor localization. 68Ga-DOTATATE PET-CT demonstrates excellent sensitivity in mapping duodenopancreatic NET disease. This modality may guide the initial workup and appears to be superior to standard somatostatin octreotide, especially for lesions smaller than 10 mm.[99,100] Many tumors are too small to be detected using standard imaging techniques, and intra-arterial secretin stimulation testing and/or intraoperative ultrasonography may also be useful.[101,102] Preoperative assessment using a combination of various biochemical and imaging modalities, intraoperative assessment of tumor burden, and resolution of hormonal hyper-secretion are critical and, in some series, have been associated with higher cure rates and longer disease-free intervals.[101-104]
In the current era of effective treatment for hyperfunctional hormone excess states, most MEN1-related deaths are due to the malignant nature of duodenopancreatic NETs. A less common but important risk of death is from malignant thymic carcinoid tumors. Indicators of a poor MEN1 prognosis include elevated fasting serum gastrin, the presence of functional hormonal syndromes, liver or distant metastases, aggressive duodenopancreatic NET growth, large duodenopancreatic NET size, or the need for multiple parathyroidectomies. The most common cause of non-MEN1–related death in this patient cohort is from cardiovascular disease.[105]
Other duodenopancreatic NETs
Glucagonomas, VIPomas, and somatostatinomas are rare but often have higher rates of malignancy than other duodenopancreatic NETs.[22] These are often treated with aggressive surgery.[106]
Insulinomas
Medical management of insulinoma using diet and medication is often unsuccessful; the mainstay of treatment for this tumor is surgical resection.[8] Insulinomas in MEN1 patients can be located throughout the pancreas, with a preponderance found in the distal gland,[107-109] and have a higher rate of metastasis than sporadic insulinoma.[106] Surgery can range from enucleation of single or multiple large tumors to partial pancreatic resection, or both,[108] to subtotal or total pancreatectomy.[107,108] More-extensive surgical approaches are associated with a lower rate of recurrence [94,95,108,110] but a higher rate of postoperative morbidity. Because insulinoma often occurs in conjunction with nonfunctioning pancreatic tumors, the selective intra-arterial calcium-injection test (SAS test) may be necessary to determine the source of insulin excess.[111] Intraoperative monitoring of insulin/glucose can help determine whether insulin-secreting tumors have been successfully excised.[102,112]
Gastrinomas
Most MEN1-associated gastrinomas originate in the duodenum. These tumors are typically multifocal and cause hyper-secretion of gastrin, with resultant peptic ulcer disease (Zollinger-Ellison syndrome).[113] The multifocal nature makes complete surgical resection difficult. It is critical to manage symptoms before considering any type of surgical intervention.[114] Historically, some groups have recommended close observation of individuals with smaller tumors (<2.0 cm on imaging) who have relief of symptoms using medications (e.g., proton pump inhibitors or histamine-2 agonists);[115] however, this approach may not be optimal for all patients.
Several published series have shown a positive correlation between primary tumor size and rate of distant metastasis. One retrospective study showed that 61% of patients with tumors larger than 3 cm had liver metastases.[24] In another series, 40% of patients with tumors larger than 3 cm had liver metastases.[116] In contrast, both of these series showed significantly lower rates of liver metastases in individuals with tumors smaller than 3 cm (32% and 4.8%, respectively). On the basis of these and other data, many groups recommend surgery in individuals with nonmetastatic gastrinoma who have tumors larger than 2 cm.[8,96]
The type of surgery for gastrinoma depends on many factors. A Whipple procedure is typically discouraged as an initial surgery, given the high postoperative morbidity and long-term complications, such as diabetes mellitus and malabsorption. Less extensive operations have been described with varying results. At a minimum, duodenectomy with intraoperative palpation and/or ultrasonography to locate and excise duodenal tumors and peri-pancreatic lymph node dissection are performed.[101,117] Because most patients with gastrinoma will have concomitant NETs throughout the pancreas, some of which may be nonfunctional, some groups recommend resection of the distal pancreas and enucleation of tumors in the pancreatic head in addition to duodenal tumor excision.[101,117,118]
Nonfunctioning NETs
Approximately 50% of individuals with MEN1 will develop nonfunctioning NETs.[21,26] These are often identified incidentally during assessment and exploration for functioning tumors. As with gastrinomas, the metastatic rate is correlated with larger tumor size.[26,86] Tumors smaller than 1.5 cm are not likely to have lymph node metastases,[119] although the presence of metastatic disease has been associated with earlier age at death than in those without duodenopancreatic NETs.[9,26]
Pituitary tumors
Medical therapy to suppress hypersecretion is often the first line of therapy for MEN1-associated pituitary tumors. In one series of 136 patients, medical therapy was successful in approximately one-half of patients with secreting tumors (49 of 116, 42%), and successful suppression was correlated with smaller tumor size.[120] Surgery is often necessary for patients who are resistant to this treatment. Radiation therapy is reserved for patients for whom complete surgical resection was not rendered.[8,121]
References
- Agarwal SK, Ozawa A, Mateo CM, et al.: The MEN1 gene and pituitary tumours. Horm Res 71 (Suppl 2): 131-8, 2009. [PUBMED Abstract]
- Trump D, Farren B, Wooding C, et al.: Clinical studies of multiple endocrine neoplasia type 1 (MEN1) QJM 89 (9): 653-69, 1996. [PUBMED Abstract]
- Chandrasekharappa SC, Guru SC, Manickam P, et al.: Positional cloning of the gene for multiple endocrine neoplasia-type 1. Science 276 (5311): 404-7, 1997. [PUBMED Abstract]
- Brandi ML, Gagel RF, Angeli A, et al.: Guidelines for diagnosis and therapy of MEN type 1 and type 2. J Clin Endocrinol Metab 86 (12): 5658-71, 2001. [PUBMED Abstract]
- Niederle B, Selberherr A, Bartsch DK, et al.: Multiple Endocrine Neoplasia Type 1 and the Pancreas: Diagnosis and Treatment of Functioning and Non-Functioning Pancreatic and Duodenal Neuroendocrine Neoplasia within the MEN1 Syndrome - An International Consensus Statement. Neuroendocrinology 111 (7): 609-630, 2021. [PUBMED Abstract]
- Carty SE, Helm AK, Amico JA, et al.: The variable penetrance and spectrum of manifestations of multiple endocrine neoplasia type 1. Surgery 124 (6): 1106-13; discussion 1113-4, 1998. [PUBMED Abstract]
- Goudet P, Dalac A, Le Bras M, et al.: MEN1 disease occurring before 21 years old: a 160-patient cohort study from the Groupe d'étude des Tumeurs Endocrines. J Clin Endocrinol Metab 100 (4): 1568-77, 2015. [PUBMED Abstract]
- Thakker RV, Newey PJ, Walls GV, et al.: Clinical practice guidelines for multiple endocrine neoplasia type 1 (MEN1). J Clin Endocrinol Metab 97 (9): 2990-3011, 2012. [PUBMED Abstract]
- Goudet P, Murat A, Binquet C, et al.: Risk factors and causes of death in MEN1 disease. A GTE (Groupe d'Etude des Tumeurs Endocrines) cohort study among 758 patients. World J Surg 34 (2): 249-55, 2010. [PUBMED Abstract]
- Chandrasekharappa SC, Teh BT: Clinical and molecular aspects of multiple endocrine neoplasia type 1. Front Horm Res 28: 50-80, 2001. [PUBMED Abstract]
- Shariq OA, Lines KE, English KA, et al.: Multiple endocrine neoplasia type 1 in children and adolescents: Clinical features and treatment outcomes. Surgery 171 (1): 77-87, 2022. [PUBMED Abstract]
- del Pozo C, García-Pascual L, Balsells M, et al.: Parathyroid carcinoma in multiple endocrine neoplasia type 1. Case report and review of the literature. Hormones (Athens) 10 (4): 326-31, 2011 Oct-Dec. [PUBMED Abstract]
- Christakis I, Busaidy NL, Cote GJ, et al.: Parathyroid carcinoma and atypical parathyroid neoplasms in MEN1 patients; A clinico-pathologic challenge. The MD Anderson case series and review of the literature. Int J Surg 31: 10-6, 2016. [PUBMED Abstract]
- Singh Ospina N, Sebo TJ, Thompson GB, et al.: Prevalence of parathyroid carcinoma in 348 patients with multiple endocrine neoplasia type 1 - case report and review of the literature. Clin Endocrinol (Oxf) 84 (2): 244-249, 2016. [PUBMED Abstract]
- Norton JA, Venzon DJ, Berna MJ, et al.: Prospective study of surgery for primary hyperparathyroidism (HPT) in multiple endocrine neoplasia-type 1 and Zollinger-Ellison syndrome: long-term outcome of a more virulent form of HPT. Ann Surg 247 (3): 501-10, 2008. [PUBMED Abstract]
- Hellman P, Skogseid B, Oberg K, et al.: Primary and reoperative parathyroid operations in hyperparathyroidism of multiple endocrine neoplasia type 1. Surgery 124 (6): 993-9, 1998. [PUBMED Abstract]
- Schreinemakers JM, Pieterman CR, Scholten A, et al.: The optimal surgical treatment for primary hyperparathyroidism in MEN1 patients: a systematic review. World J Surg 35 (9): 1993-2005, 2011. [PUBMED Abstract]
- Christakis I, Qiu W, Hyde SM, et al.: Genotype-phenotype pancreatic neuroendocrine tumor relationship in multiple endocrine neoplasia type 1 patients: A 23-year experience at a single institution. Surgery 163 (1): 212-217, 2018. [PUBMED Abstract]
- Donegan D, Singh Ospina N, Rodriguez-Gutierrez R, et al.: Long-term outcomes in patients with multiple endocrine neoplasia type 1 and pancreaticoduodenal neuroendocrine tumours. Clin Endocrinol (Oxf) 86 (2): 199-206, 2017. [PUBMED Abstract]
- Norton JA, Krampitz G, Jensen RT: Multiple Endocrine Neoplasia: Genetics and Clinical Management. Surg Oncol Clin N Am 24 (4): 795-832, 2015. [PUBMED Abstract]
- Thomas-Marques L, Murat A, Delemer B, et al.: Prospective endoscopic ultrasonographic evaluation of the frequency of nonfunctioning pancreaticoduodenal endocrine tumors in patients with multiple endocrine neoplasia type 1. Am J Gastroenterol 101 (2): 266-73, 2006. [PUBMED Abstract]
- Lévy-Bohbot N, Merle C, Goudet P, et al.: Prevalence, characteristics and prognosis of MEN 1-associated glucagonomas, VIPomas, and somatostatinomas: study from the GTE (Groupe des Tumeurs Endocrines) registry. Gastroenterol Clin Biol 28 (11): 1075-81, 2004. [PUBMED Abstract]
- Pipeleers-Marichal M, Somers G, Willems G, et al.: Gastrinomas in the duodenums of patients with multiple endocrine neoplasia type 1 and the Zollinger-Ellison syndrome. N Engl J Med 322 (11): 723-7, 1990. [PUBMED Abstract]
- Weber HC, Venzon DJ, Lin JT, et al.: Determinants of metastatic rate and survival in patients with Zollinger-Ellison syndrome: a prospective long-term study. Gastroenterology 108 (6): 1637-49, 1995. [PUBMED Abstract]
- Tonelli F, Giudici F, Fratini G, et al.: Pancreatic endocrine tumors in multiple endocrine neoplasia type 1 syndrome: review of literature. Endocr Pract 17 (Suppl 3): 33-40, 2011 Jul-Aug. [PUBMED Abstract]
- Triponez F, Dosseh D, Goudet P, et al.: Epidemiology data on 108 MEN 1 patients from the GTE with isolated nonfunctioning tumors of the pancreas. Ann Surg 243 (2): 265-72, 2006. [PUBMED Abstract]
- Corbetta S, Pizzocaro A, Peracchi M, et al.: Multiple endocrine neoplasia type 1 in patients with recognized pituitary tumours of different types. Clin Endocrinol (Oxf) 47 (5): 507-12, 1997. [PUBMED Abstract]
- Darling TN, Skarulis MC, Steinberg SM, et al.: Multiple facial angiofibromas and collagenomas in patients with multiple endocrine neoplasia type 1. Arch Dermatol 133 (7): 853-7, 1997. [PUBMED Abstract]
- Ventura M, Melo M, Carrilho F: Outcome and long-term follow-up of adrenal lesions in multiple endocrine neoplasia type 1. Arch Endocrinol Metab 63 (5): 516-523, 2019. [PUBMED Abstract]
- Machens A, Schaaf L, Karges W, et al.: Age-related penetrance of endocrine tumours in multiple endocrine neoplasia type 1 (MEN1): a multicentre study of 258 gene carriers. Clin Endocrinol (Oxf) 67 (4): 613-22, 2007. [PUBMED Abstract]
- Pieterman CR, Schreinemakers JM, Koppeschaar HP, et al.: Multiple endocrine neoplasia type 1 (MEN1): its manifestations and effect of genetic screening on clinical outcome. Clin Endocrinol (Oxf) 70 (4): 575-81, 2009. [PUBMED Abstract]
- Waldmann J, Bartsch DK, Kann PH, et al.: Adrenal involvement in multiple endocrine neoplasia type 1: results of 7 years prospective screening. Langenbecks Arch Surg 392 (4): 437-43, 2007. [PUBMED Abstract]
- Gibril F, Schumann M, Pace A, et al.: Multiple endocrine neoplasia type 1 and Zollinger-Ellison syndrome: a prospective study of 107 cases and comparison with 1009 cases from the literature. Medicine (Baltimore) 83 (1): 43-83, 2004. [PUBMED Abstract]
- McKeeby JL, Li X, Zhuang Z, et al.: Multiple leiomyomas of the esophagus, lung, and uterus in multiple endocrine neoplasia type 1. Am J Pathol 159 (3): 1121-7, 2001. [PUBMED Abstract]
- Vortmeyer AO, Lubensky IA, Skarulis M, et al.: Multiple endocrine neoplasia type 1: atypical presentation, clinical course, and genetic analysis of multiple tumors. Mod Pathol 12 (9): 919-24, 1999. [PUBMED Abstract]
- Yamazaki M, Suzuki S, Kosugi S, et al.: Delay in the diagnosis of multiple endocrine neoplasia type 1: typical symptoms are frequently overlooked. Endocr J 59 (9): 797-807, 2012. [PUBMED Abstract]
- Lourenço DM, Toledo RA, Coutinho FL, et al.: The impact of clinical and genetic screenings on the management of the multiple endocrine neoplasia type 1. Clinics (Sao Paulo) 62 (4): 465-76, 2007. [PUBMED Abstract]
- van Leeuwaarde RS, van Nesselrooij BP, Hermus AR, et al.: Impact of Delay in Diagnosis in Outcomes in MEN1: Results From the Dutch MEN1 Study Group. J Clin Endocrinol Metab 101 (3): 1159-65, 2016. [PUBMED Abstract]
- Strømsvik N, Nordin K, Berglund G, et al.: Living with multiple endocrine neoplasia type 1: decent care-insufficient medical and genetic information: a qualitative study of MEN 1 patients in a Swedish hospital. J Genet Couns 16 (1): 105-17, 2007. [PUBMED Abstract]
- Marini F, Giusti F, Tonelli F, et al.: Management impact: effects on quality of life and prognosis in MEN1. Endocr Relat Cancer 24 (10): T227-T242, 2017. [PUBMED Abstract]
- Roy PK, Venzon DJ, Shojamanesh H, et al.: Zollinger-Ellison syndrome. Clinical presentation in 261 patients. Medicine (Baltimore) 79 (6): 379-411, 2000. [PUBMED Abstract]
- Bardram L, Stage JG: Frequency of endocrine disorders in patients with the Zollinger-Ellison syndrome. Scand J Gastroenterol 20 (2): 233-8, 1985. [PUBMED Abstract]
- Uchino S, Noguchi S, Sato M, et al.: Screening of the Men1 gene and discovery of germ-line and somatic mutations in apparently sporadic parathyroid tumors. Cancer Res 60 (19): 5553-7, 2000. [PUBMED Abstract]
- Scheithauer BW, Laws ER, Kovacs K, et al.: Pituitary adenomas of the multiple endocrine neoplasia type I syndrome. Semin Diagn Pathol 4 (3): 205-11, 1987. [PUBMED Abstract]
- Newey PJ, Thakker RV: Role of multiple endocrine neoplasia type 1 mutational analysis in clinical practice. Endocr Pract 17 (Suppl 3): 8-17, 2011 Jul-Aug. [PUBMED Abstract]
- Hampel H, Bennett RL, Buchanan A, et al.: A practice guideline from the American College of Medical Genetics and Genomics and the National Society of Genetic Counselors: referral indications for cancer predisposition assessment. Genet Med 17 (1): 70-87, 2015. [PUBMED Abstract]
- Bashford MT, Kohlman W, Everett J, et al.: Addendum: A practice guideline from the American College of Medical Genetics and Genomics and the National Society of Genetic Counselors: referral indications for cancer predisposition assessment. Genet Med 21 (12): 2844, 2019. [PUBMED Abstract]
- Larsson C, Skogseid B, Oberg K, et al.: Multiple endocrine neoplasia type 1 gene maps to chromosome 11 and is lost in insulinoma. Nature 332 (6159): 85-7, 1988. [PUBMED Abstract]
- Bassett JH, Forbes SA, Pannett AA, et al.: Characterization of mutations in patients with multiple endocrine neoplasia type 1. Am J Hum Genet 62 (2): 232-44, 1998. [PUBMED Abstract]
- Lemos MC, Thakker RV: Multiple endocrine neoplasia type 1 (MEN1): analysis of 1336 mutations reported in the first decade following identification of the gene. Hum Mutat 29 (1): 22-32, 2008. [PUBMED Abstract]
- Concolino P, Costella A, Capoluongo E: Multiple endocrine neoplasia type 1 (MEN1): An update of 208 new germline variants reported in the last nine years. Cancer Genet 209 (1-2): 36-41, 2016 Jan-Feb. [PUBMED Abstract]
- Brandi ML, Agarwal SK, Perrier ND, et al.: Multiple Endocrine Neoplasia Type 1: Latest Insights. Endocr Rev 42 (2): 133-170, 2021. [PUBMED Abstract]
- Giraud S, Zhang CX, Serova-Sinilnikova O, et al.: Germ-line mutation analysis in patients with multiple endocrine neoplasia type 1 and related disorders. Am J Hum Genet 63 (2): 455-67, 1998. [PUBMED Abstract]
- Wautot V, Vercherat C, Lespinasse J, et al.: Germline mutation profile of MEN1 in multiple endocrine neoplasia type 1: search for correlation between phenotype and the functional domains of the MEN1 protein. Hum Mutat 20 (1): 35-47, 2002. [PUBMED Abstract]
- van den Broek MFM, van Nesselrooij BPM, Pieterman CRC, et al.: Clues For Genetic Anticipation In Multiple Endocrine Neoplasia Type 1. J Clin Endocrinol Metab 105 (7): , 2020. [PUBMED Abstract]
- Thevenon J, Bourredjem A, Faivre L, et al.: Unraveling the intrafamilial correlations and heritability of tumor types in MEN1: a Groupe d'étude des Tumeurs Endocrines study. Eur J Endocrinol 173 (6): 819-26, 2015. [PUBMED Abstract]
- Agarwal SK, Kester MB, Debelenko LV, et al.: Germline mutations of the MEN1 gene in familial multiple endocrine neoplasia type 1 and related states. Hum Mol Genet 6 (7): 1169-75, 1997. [PUBMED Abstract]
- Klein RD, Salih S, Bessoni J, et al.: Clinical testing for multiple endocrine neoplasia type 1 in a DNA diagnostic laboratory. Genet Med 7 (2): 131-8, 2005. [PUBMED Abstract]
- Teh BT, Farnebo F, Kristoffersson U, et al.: Autosomal dominant primary hyperparathyroidism and jaw tumor syndrome associated with renal hamartomas and cystic kidney disease: linkage to 1q21-q32 and loss of the wild type allele in renal hamartomas. J Clin Endocrinol Metab 81 (12): 4204-11, 1996. [PUBMED Abstract]
- Carpten JD, Robbins CM, Villablanca A, et al.: HRPT2, encoding parafibromin, is mutated in hyperparathyroidism-jaw tumor syndrome. Nat Genet 32 (4): 676-80, 2002. [PUBMED Abstract]
- Marx SJ: Multiple endocrine neoplasia type 1. In: Vogelstein B, Kinzler KW, eds.: The Genetic Basis of Human Cancer. McGraw-Hill, 1998, pp 489-506.
- Warner J, Epstein M, Sweet A, et al.: Genetic testing in familial isolated hyperparathyroidism: unexpected results and their implications. J Med Genet 41 (3): 155-60, 2004. [PUBMED Abstract]
- Mizusawa N, Uchino S, Iwata T, et al.: Genetic analyses in patients with familial isolated hyperparathyroidism and hyperparathyroidism-jaw tumour syndrome. Clin Endocrinol (Oxf) 65 (1): 9-16, 2006. [PUBMED Abstract]
- Cetani F, Pardi E, Borsari S, et al.: Molecular pathogenesis of primary hyperparathyroidism. J Endocrinol Invest 34 (7 Suppl): 35-9, 2011. [PUBMED Abstract]
- Miedlich S, Lohmann T, Schneyer U, et al.: Familial isolated primary hyperparathyroidism--a multiple endocrine neoplasia type 1 variant? Eur J Endocrinol 145 (2): 155-60, 2001. [PUBMED Abstract]
- Cetani F, Pardi E, Ambrogini E, et al.: Genetic analyses in familial isolated hyperparathyroidism: implication for clinical assessment and surgical management. Clin Endocrinol (Oxf) 64 (2): 146-52, 2006. [PUBMED Abstract]
- Raue F, Frank-Raue K: Primary hyperparathyroidism--what the nephrologist should know--an update. Nephrol Dial Transplant 22 (3): 696-9, 2007. [PUBMED Abstract]
- Romero Arenas MA, Morris LF, Rich TA, et al.: Preoperative multiple endocrine neoplasia type 1 diagnosis improves the surgical outcomes of pediatric patients with primary hyperparathyroidism. J Pediatr Surg 49 (4): 546-50, 2014. [PUBMED Abstract]
- Thakker RV: Multiple endocrine neoplasia type 1 (MEN1) and type 4 (MEN4). Mol Cell Endocrinol 386 (1-2): 2-15, 2014. [PUBMED Abstract]
- Christensen SE, Nissen PH, Vestergaard P, et al.: Familial hypocalciuric hypercalcaemia: a review. Curr Opin Endocrinol Diabetes Obes 18 (6): 359-70, 2011. [PUBMED Abstract]
- Nesbit MA, Hannan FM, Howles SA, et al.: Mutations affecting G-protein subunit α11 in hypercalcemia and hypocalcemia. N Engl J Med 368 (26): 2476-2486, 2013. [PUBMED Abstract]
- Nesbit MA, Hannan FM, Howles SA, et al.: Mutations in AP2S1 cause familial hypocalciuric hypercalcemia type 3. Nat Genet 45 (1): 93-7, 2013. [PUBMED Abstract]
- Mennetrey C, Le Bras M, Bando-Delaunay A, et al.: Value of Somatostatin Receptor PET/CT in Patients With MEN1 at Various Stages of Their Disease. J Clin Endocrinol Metab 107 (5): e2056-e2064, 2022. [PUBMED Abstract]
- So A, Pointon O, Hodgson R, et al.: An assessment of 18 F-FDG PET/CT for thoracic screening and risk stratification of pulmonary nodules in multiple endocrine neoplasia type 1. Clin Endocrinol (Oxf) 88 (5): 683-691, 2018. [PUBMED Abstract]
- van den Broek MFM, de Laat JM, van Leeuwaarde RS, et al.: The Management of Neuroendocrine Tumors of the Lung in MEN1: Results From the Dutch MEN1 Study Group. J Clin Endocrinol Metab 106 (2): e1014-e1027, 2021. [PUBMED Abstract]
- Langer P, Kann PH, Fendrich V, et al.: Prospective evaluation of imaging procedures for the detection of pancreaticoduodenal endocrine tumors in patients with multiple endocrine neoplasia type 1. World J Surg 28 (12): 1317-22, 2004. [PUBMED Abstract]
- de Laat JM, Dekkers OM, Pieterman CR, et al.: Long-Term Natural Course of Pituitary Tumors in Patients With MEN1: Results From the DutchMEN1 Study Group (DMSG). J Clin Endocrinol Metab 100 (9): 3288-96, 2015. [PUBMED Abstract]
- Shirali AS, Pieterman CRC, Lewis MA, et al.: It's not a mystery, it's in the history: Multidisciplinary management of multiple endocrine neoplasia type 1. CA Cancer J Clin 71 (5): 369-380, 2021. [PUBMED Abstract]
- Lee ME, Ortega-Sustache YM, Agarwal SK, et al.: Patients With MEN1 Are at an Increased Risk for Venous Thromboembolism. J Clin Endocrinol Metab 106 (2): e460-e468, 2021. [PUBMED Abstract]
- Pieterman CR, van Hulsteijn LT, den Heijer M, et al.: Primary hyperparathyroidism in MEN1 patients: a cohort study with longterm follow-up on preferred surgical procedure and the relation with genotype. Ann Surg 255 (6): 1171-8, 2012. [PUBMED Abstract]
- Nilubol N, Weinstein LS, Simonds WF, et al.: Limited Parathyroidectomy in Multiple Endocrine Neoplasia Type 1-Associated Primary Hyperparathyroidism: A Setup for Failure. Ann Surg Oncol 23 (2): 416-23, 2016. [PUBMED Abstract]
- Lairmore TC, Govednik CM, Quinn CE, et al.: A randomized, prospective trial of operative treatments for hyperparathyroidism in patients with multiple endocrine neoplasia type 1. Surgery 156 (6): 1326-34; discussion 1334-5, 2014. [PUBMED Abstract]
- Landry JP, Pieterman CRC, Clemente-Gutierrez U, et al.: Evaluation of risk factors, long-term outcomes, and immediate and delayed autotransplantation to minimize postsurgical hypoparathyroidism in multiple endocrine neoplasia type 1 (MEN1): A retrospective cohort study. Surgery 171 (5): 1240-1246, 2022. [PUBMED Abstract]
- Ratnayake CBB, Loveday BP, Windsor JA, et al.: Patient characteristics and clinical outcomes following initial surgical intervention for MEN1 associated pancreatic neuroendocrine tumours: A systematic review and exploratory meta-analysis of the literature. Pancreatology 19 (3): 462-471, 2019. [PUBMED Abstract]
- Kishi Y, Shimada K, Nara S, et al.: Basing treatment strategy for non-functional pancreatic neuroendocrine tumors on tumor size. Ann Surg Oncol 21 (9): 2882-8, 2014. [PUBMED Abstract]
- Nell S, Verkooijen HM, Pieterman CRC, et al.: Management of MEN1 Related Nonfunctioning Pancreatic NETs: A Shifting Paradigm: Results From the DutchMEN1 Study Group. Ann Surg 267 (6): 1155-1160, 2018. [PUBMED Abstract]
- Qiu W, Christakis I, Silva A, et al.: Utility of chromogranin A, pancreatic polypeptide, glucagon and gastrin in the diagnosis and follow-up of pancreatic neuroendocrine tumours in multiple endocrine neoplasia type 1 patients. Clin Endocrinol (Oxf) 85 (3): 400-7, 2016. [PUBMED Abstract]
- Ramundo V, Del Prete M, Marotta V, et al.: Impact of long-acting octreotide in patients with early-stage MEN1-related duodeno-pancreatic neuroendocrine tumours. Clin Endocrinol (Oxf) 80 (6): 850-5, 2014. [PUBMED Abstract]
- Triponez F, Goudet P, Dosseh D, et al.: Is surgery beneficial for MEN1 patients with small (< or = 2 cm), nonfunctioning pancreaticoduodenal endocrine tumor? An analysis of 65 patients from the GTE. World J Surg 30 (5): 654-62; discussion 663-4, 2006. [PUBMED Abstract]
- Bettini R, Partelli S, Boninsegna L, et al.: Tumor size correlates with malignancy in nonfunctioning pancreatic endocrine tumor. Surgery 150 (1): 75-82, 2011. [PUBMED Abstract]
- Triponez F, Sadowski SM, Pattou F, et al.: Long-term Follow-up of MEN1 Patients Who Do Not Have Initial Surgery for Small ≤2 cm Nonfunctioning Pancreatic Neuroendocrine Tumors, an AFCE and GTE Study: Association Francophone de Chirurgie Endocrinienne & Groupe d'Etude des Tumeurs Endocrines. Ann Surg 268 (1): 158-164, 2018. [PUBMED Abstract]
- Kornaczewski Jackson ER, Pointon OP, Bohmer R, et al.: Utility of FDG-PET Imaging for Risk Stratification of Pancreatic Neuroendocrine Tumors in MEN1. J Clin Endocrinol Metab 102 (6): 1926-1933, 2017. [PUBMED Abstract]
- Brunner SM, Weber F, Werner JM, et al.: Neuroendocrine tumors of the pancreas: a retrospective single-center analysis using the ENETS TNM-classification and immunohistochemical markers for risk stratification. BMC Surg 15: 49, 2015. [PUBMED Abstract]
- Bartsch DK, Langer P, Wild A, et al.: Pancreaticoduodenal endocrine tumors in multiple endocrine neoplasia type 1: surgery or surveillance? Surgery 128 (6): 958-66, 2000. [PUBMED Abstract]
- Bartsch DK, Fendrich V, Langer P, et al.: Outcome of duodenopancreatic resections in patients with multiple endocrine neoplasia type 1. Ann Surg 242 (6): 757-64, discussion 764-6, 2005. [PUBMED Abstract]
- Norton JA, Jensen RT: Role of surgery in Zollinger-Ellison syndrome. J Am Coll Surg 205 (4 Suppl): S34-7, 2007. [PUBMED Abstract]
- Lopez CL, Waldmann J, Fendrich V, et al.: Long-term results of surgery for pancreatic neuroendocrine neoplasms in patients with MEN1. Langenbecks Arch Surg 396 (8): 1187-96, 2011. [PUBMED Abstract]
- Drymousis P, Raptis DA, Spalding D, et al.: Laparoscopic versus open pancreas resection for pancreatic neuroendocrine tumours: a systematic review and meta-analysis. HPB (Oxford) 16 (5): 397-406, 2014. [PUBMED Abstract]
- Morgat C, Vélayoudom-Céphise FL, Schwartz P, et al.: Evaluation of (68)Ga-DOTA-TOC PET/CT for the detection of duodenopancreatic neuroendocrine tumors in patients with MEN1. Eur J Nucl Med Mol Imaging 43 (7): 1258-66, 2016. [PUBMED Abstract]
- Lastoria S, Marciello F, Faggiano A, et al.: Role of (68)Ga-DOTATATE PET/CT in patients with multiple endocrine neoplasia type 1 (MEN1). Endocrine 52 (3): 488-94, 2016. [PUBMED Abstract]
- Imamura M, Komoto I, Ota S, et al.: Biochemically curative surgery for gastrinoma in multiple endocrine neoplasia type 1 patients. World J Gastroenterol 17 (10): 1343-53, 2011. [PUBMED Abstract]
- Tonelli F, Fratini G, Nesi G, et al.: Pancreatectomy in multiple endocrine neoplasia type 1-related gastrinomas and pancreatic endocrine neoplasias. Ann Surg 244 (1): 61-70, 2006. [PUBMED Abstract]
- Lewis MA, Thompson GB, Young WF: Preoperative assessment of the pancreas in multiple endocrine neoplasia type 1. World J Surg 36 (6): 1375-81, 2012. [PUBMED Abstract]
- van Asselt SJ, Brouwers AH, van Dullemen HM, et al.: EUS is superior for detection of pancreatic lesions compared with standard imaging in patients with multiple endocrine neoplasia type 1. Gastrointest Endosc 81 (1): 159-167.e2, 2015. [PUBMED Abstract]
- Ito T, Igarashi H, Uehara H, et al.: Causes of death and prognostic factors in multiple endocrine neoplasia type 1: a prospective study: comparison of 106 MEN1/Zollinger-Ellison syndrome patients with 1613 literature MEN1 patients with or without pancreatic endocrine tumors. Medicine (Baltimore) 92 (3): 135-81, 2013. [PUBMED Abstract]
- Akerström G, Stålberg P: Surgical management of MEN-1 and -2: state of the art. Surg Clin North Am 89 (5): 1047-68, 2009. [PUBMED Abstract]
- O'Riordain DS, O'Brien T, van Heerden JA, et al.: Surgical management of insulinoma associated with multiple endocrine neoplasia type I. World J Surg 18 (4): 488-93; discussion 493-4, 1994 Jul-Aug. [PUBMED Abstract]
- Crippa S, Zerbi A, Boninsegna L, et al.: Surgical management of insulinomas: short- and long-term outcomes after enucleations and pancreatic resections. Arch Surg 147 (3): 261-6, 2012. [PUBMED Abstract]
- Sakurai A, Yamazaki M, Suzuki S, et al.: Clinical features of insulinoma in patients with multiple endocrine neoplasia type 1: analysis of the database of the MEN Consortium of Japan. Endocr J 59 (10): 859-66, 2012. [PUBMED Abstract]
- Vezzosi D, Cardot-Bauters C, Bouscaren N, et al.: Long-term results of the surgical management of insulinoma patients with MEN1: a Groupe d'étude des Tumeurs Endocrines (GTE) retrospective study. Eur J Endocrinol 172 (3): 309-19, 2015. [PUBMED Abstract]
- Grant CS: Insulinoma. Best Pract Res Clin Gastroenterol 19 (5): 783-98, 2005. [PUBMED Abstract]
- Giudici F, Nesi G, Brandi ML, et al.: Surgical management of insulinomas in multiple endocrine neoplasia type 1. Pancreas 41 (4): 547-53, 2012. [PUBMED Abstract]
- Plöckinger U: Diagnosis and Treatment of Gastrinomas in Multiple Endocrine Neoplasia Type 1 (MEN-1). Cancers (Basel) 4 (1): 39-54, 2012. [PUBMED Abstract]
- Falconi M, Eriksson B, Kaltsas G, et al.: ENETS Consensus Guidelines Update for the Management of Patients with Functional Pancreatic Neuroendocrine Tumors and Non-Functional Pancreatic Neuroendocrine Tumors. Neuroendocrinology 103 (2): 153-71, 2016. [PUBMED Abstract]
- Mignon M, Cadiot G: Diagnostic and therapeutic criteria in patients with Zollinger-Ellison syndrome and multiple endocrine neoplasia type 1. J Intern Med 243 (6): 489-94, 1998. [PUBMED Abstract]
- Cadiot G, Vuagnat A, Doukhan I, et al.: Prognostic factors in patients with Zollinger-Ellison syndrome and multiple endocrine neoplasia type 1. Groupe d'Etude des Néoplasies Endocriniennes Multiples (GENEM and groupe de Recherche et d'Etude du Syndrome de Zollinger-Ellison (GRESZE). Gastroenterology 116 (2): 286-93, 1999. [PUBMED Abstract]
- Dickson PV, Rich TA, Xing Y, et al.: Achieving eugastrinemia in MEN1 patients: both duodenal inspection and formal lymph node dissection are important. Surgery 150 (6): 1143-52, 2011. [PUBMED Abstract]
- Akerström G, Stålberg P, Hellman P: Surgical management of pancreatico-duodenal tumors in multiple endocrine neoplasia syndrome type 1. Clinics (Sao Paulo) 67 (Suppl 1): 173-8, 2012. [PUBMED Abstract]
- Zhang IY, Zhao J, Fernandez-Del Castillo C, et al.: Operative Versus Nonoperative Management of Nonfunctioning Pancreatic Neuroendocrine Tumors. J Gastrointest Surg 20 (2): 277-83, 2016. [PUBMED Abstract]
- Vergès B, Boureille F, Goudet P, et al.: Pituitary disease in MEN type 1 (MEN1): data from the France-Belgium MEN1 multicenter study. J Clin Endocrinol Metab 87 (2): 457-65, 2002. [PUBMED Abstract]
- Pieterman CR, Vriens MR, Dreijerink KM, et al.: Care for patients with multiple endocrine neoplasia type 1: the current evidence base. Fam Cancer 10 (1): 157-71, 2011. [PUBMED Abstract]
Multiple Endocrine Neoplasia Type 2
Multiple endocrine neoplasia type 2 (MEN2) is caused by pathogenic variants in the RET gene. The endocrine disorders observed in MEN2 include medullary thyroid cancer and its precursor, C-cell hyperplasia (referred to as C-cell neoplasia or C-cell carcinoma in situ in more recent publications);[1] pheochromocytoma; and parathyroid adenomas and/or hyperplasia. For more information about MEN2, see Multiple Endocrine Neoplasia Type 2.
References
- Wells SA, Asa SL, Dralle H, et al.: Revised American Thyroid Association guidelines for the management of medullary thyroid carcinoma. Thyroid 25 (6): 567-610, 2015. [PUBMED Abstract]
Multiple Endocrine Neoplasia Type 4
Introduction
Multiple endocrine neoplasia type 4 (MEN4) is a novel, rare syndrome with clinical features that overlap with the other MEN syndromes. The most common phenotype of the 19 established cases of MEN4 that have been described to date is primary hyperparathyroidism (PHPT), followed by pituitary adenomas. MEN4 is caused by germline pathogenic variants in the tumor suppressor gene CDKN1B (12p13.1).[1] This syndrome was discovered initially in rats (MENX) [2] and later in humans (MEN4). The syndrome has the phenotype of being multiple endocrine neoplasia type 1 (MEN1)-like. The incidence of CDKN1B variants in patients with an MEN1-related phenotype is difficult to estimate, but it is likely to be in the range of 1.5% to 3.7%.[3-5] Pathogenic variants leading to the MEN4 phenotype are transmitted in an autosomal dominant fashion.
Clinical Diagnosis
PHPT due to parathyroid neoplasia affects approximately 80% of the reported cases of MEN4. PHPT occurs at a later age in MEN4 than in MEN1 (mean age ~56 y vs. ~25 y, respectively), with a female predominance.[6] There have been no reports of PHPT recurrence after surgical resection, which might indicate that PHPT in MEN4 represents an overall milder disease spectrum than in MEN1. Pituitary involvement in MEN4 is the second most common manifestation of the disease, affecting approximately 37% of the reported cases. Pituitary adenomas in MEN4 vary and include nonfunctional, somatotropinoma, prolactinoma, or corticotropinoma types. The age at diagnosis for these lesions also varies widely, from 30 years to 79 years. The youngest patient reported to have MEN4 presented at age 30 years with acromegaly.[2] Pancreatic neuroendocrine tumors (NETs) have been rare, with only a few cases reported. These include duodenopancreatic or gastrointestinal NETs that could be nonfunctioning or hormonally active and may secrete several substances, including gastrin, insulin, adrenocorticotropic hormone, or vasoactive intestinal polypeptide. Although adrenal neoplasia is a frequent finding in MEN1, only one case of nonfunctional bilateral adrenal nodules has been reported in MEN4.[5] Skin manifestations that are commonly reported in MEN1, such as lipomas, angiofibromas, and collagenomas, have not been reported in MEN4. There is no known genotype-phenotype correlation.
Genetics, Inheritance, and Genetic Testing for MEN4
The CDKN1B variant codes for p27Kip1 (commonly referred to as p27 or KIP1), a putative tumor suppressor gene that regulates cell cycle progression. Alterations in this gene lead to a decrease in expression of p27 protein, triggering uncontrolled cell cycle progression. Although the loss of one allele of p27 is a frequent event in many human cancers, the remaining allele is rarely mutated or lost by loss of heterozygosity in human cancers.[7] Somatic variants or germline pathogenic variants in CDKN1B have also been identified in patients with sporadic PHPT, small intestinal NETs, lymphoma, and breast cancer. These findings demonstrate a novel role for CDKN1B as a tumor susceptibility gene in other neoplasms.[8-10]
To date, only 19 cases having CDKN1B germline variants have been reported in the medical literature.[8] Thirteen pathogenic germline variants that have been frameshift, nonsense, or missense variants have been described.[11,12]
Index cases or individuals with MEN1-like features and negative results of MEN1 genetic testing are offered genetic counseling and testing for MEN4. Confirmation of an MEN4 diagnosis is only made with genetic testing for CDKN1B variants. In clinical practice, patients with asymptomatic or symptomatic PHPT who are also young (typically <30 y) and have multigland disease, parathyroid carcinoma, or atypical adenoma, or those with a family history or evidence of syndromic disease and negative for MEN1 or RET, are candidates for genetic testing for CDKN1B using accredited laboratories.[8] For those with proven disease, screening is also offered to a first-degree relative with or without MEN1 features. The identification of a germline CDKN1B variant should prompt periodic clinical biochemical screening for MEN4.
Surveillance
Surveillance of CDKN1B pathogenic variant carriers should be performed, though guidelines have not been established yet.[8,13] Currently, surveillance is mainly clinical and focuses on finding an excess of growth hormone. It is recommended that annual biochemical testing for insulin-like growth factor-1 and annual blood work be done to assess for PHPT.[13] For known CDKN1B carriers, surveillance begins at adolescence. The role of imaging has not been established.
Interventions
Similar to the treatment used in other familial syndromes, surgical treatment is recommended for parathyroid and pituitary disease. For more information, see the MEN1 section.
Outcomes
A study of 293 MEN1 pathogenic variant–positive cases and 30 MEN1 pathogenic variant–negative cases, all with the MEN1 phenotype, showed that the pathogenic variant–negative cohort developed disease manifestations later in life, with improved life expectancy.[14] One of the limitations in applying this finding to MEN4 is that only 1 of these 30 MEN1-negative patients was CDKN1B positive.
References
- Marinoni I, Pellegata NS: p27kip1: a new multiple endocrine neoplasia gene? Neuroendocrinology 93 (1): 19-28, 2011. [PUBMED Abstract]
- Pellegata NS, Quintanilla-Martinez L, Siggelkow H, et al.: Germ-line mutations in p27Kip1 cause a multiple endocrine neoplasia syndrome in rats and humans. Proc Natl Acad Sci U S A 103 (42): 15558-63, 2006. [PUBMED Abstract]
- Georgitsi M, Raitila A, Karhu A, et al.: Germline CDKN1B/p27Kip1 mutation in multiple endocrine neoplasia. J Clin Endocrinol Metab 92 (8): 3321-5, 2007. [PUBMED Abstract]
- Agarwal SK, Mateo CM, Marx SJ: Rare germline mutations in cyclin-dependent kinase inhibitor genes in multiple endocrine neoplasia type 1 and related states. J Clin Endocrinol Metab 94 (5): 1826-34, 2009. [PUBMED Abstract]
- Molatore S, Marinoni I, Lee M, et al.: A novel germline CDKN1B mutation causing multiple endocrine tumors: clinical, genetic and functional characterization. Hum Mutat 31 (11): E1825-35, 2010. [PUBMED Abstract]
- Lee M, Pellegata NS: Multiple endocrine neoplasia type 4. Front Horm Res 41: 63-78, 2013. [PUBMED Abstract]
- Philipp-Staheli J, Payne SR, Kemp CJ: p27(Kip1): regulation and function of a haploinsufficient tumor suppressor and its misregulation in cancer. Exp Cell Res 264 (1): 148-68, 2001. [PUBMED Abstract]
- Alrezk R, Hannah-Shmouni F, Stratakis CA: MEN4 and CDKN1B mutations: the latest of the MEN syndromes. Endocr Relat Cancer 24 (10): T195-T208, 2017. [PUBMED Abstract]
- Malanga D, De Gisi S, Riccardi M, et al.: Functional characterization of a rare germline mutation in the gene encoding the cyclin-dependent kinase inhibitor p27Kip1 (CDKN1B) in a Spanish patient with multiple endocrine neoplasia-like phenotype. Eur J Endocrinol 166 (3): 551-60, 2012. [PUBMED Abstract]
- Occhi G, Regazzo D, Trivellin G, et al.: A novel mutation in the upstream open reading frame of the CDKN1B gene causes a MEN4 phenotype. PLoS Genet 9 (3): e1003350, 2013. [PUBMED Abstract]
- Georgitsi M: MEN-4 and other multiple endocrine neoplasias due to cyclin-dependent kinase inhibitors (p27(Kip1) and p18(INK4C)) mutations. Best Pract Res Clin Endocrinol Metab 24 (3): 425-37, 2010. [PUBMED Abstract]
- Lee M, Pellegata NS: Multiple endocrine neoplasia syndromes associated with mutation of p27. J Endocrinol Invest 36 (9): 781-7, 2013. [PUBMED Abstract]
- Wasserman JD, Tomlinson GE, Druker H, et al.: Multiple Endocrine Neoplasia and Hyperparathyroid-Jaw Tumor Syndromes: Clinical Features, Genetics, and Surveillance Recommendations in Childhood. Clin Cancer Res 23 (13): e123-e132, 2017. [PUBMED Abstract]
- de Laat JM, van der Luijt RB, Pieterman CR, et al.: MEN1 redefined, a clinical comparison of mutation-positive and mutation-negative patients. BMC Med 14 (1): 182, 2016. [PUBMED Abstract]
Familial Pheochromocytoma and Paraganglioma Syndrome
Introduction
Paragangliomas (PGLs) and pheochromocytomas (PHEOs) are rare tumors arising from chromaffin cells, which have the ability to synthesize, store, and secrete catecholamines and neuropeptides. Individuals may present with secondary hypertension. In 2004, the World Health Organization characterized adrenal gland tumors as PHEOs.[1] The term paraganglioma is reserved for non-adrenal (or extra-adrenal) neoplasms and may arise in various sites from the paraganglia along the parasympathetic nerves or the sympathetic trunk. PGLs may be found in the head and neck region, abdomen, or pelvis. Only those arising from sympathetic neural chains have secretory capacity. PGLs found in the skull base or head and neck region typically arise in the glomus cells, near the carotid body, along the vagal nerve or jugular fosse, and are usually from parasympathetic paraganglia and therefore rarely secrete catecholamines.[2,3] The most recognizable tumors are found at the carotid body. PGLs below the neck are most commonly located in the upper mediastinum or the urinary bladder.[3] The reported incidence of these tumors in the general population is variable because they may be asymptomatic but ranges from 1 in 30,000 to 1 in 100,000 individuals.[3] One autopsy study found a much greater incidence of 1 in 2,000 individuals, suggesting a high frequency of occult tumors.[4] PGLs have an equal sex distribution. They can occur at any age but have the highest incidence between the ages of 40 and 50 years.[5,6]
Clinical Description
PHEOs and PGLs may occur sporadically, as manifestations of a hereditary syndrome, or as the sole tumor in one of several hereditary PHEO/PGL syndromes. Some individuals with a predisposition to PHEOs/PGLs do not have a known family history of these tumors. For example, a study of 108 patients with PHEOs/PGLs found that 33% of patients with a germline pathogenic variant did not have family histories of a hereditary PHEO/PGL syndrome. Similarly, 36% of the patients with SDHB germline pathogenic variants did not have family histories of PHEOs/PGLs or personal histories of PHEOs/PGLs at presentation.[7]
Most sporadic PHEOs occur unilaterally. Bilateral PHEOs are more likely to occur in a hereditary condition. A single-center study of patients with PHEOs found that up to 7% of adults and 37.5% of children had bilateral PHEOs. Synchronous tumors were seen in 80% of patients with bilateral PHEOs. When metachronous PHEOs were identified, the median time to develop a second PHEO was 4.5 years (range 1–38 y). Hereditary cancer syndromes were identified in 80% of individuals with bilateral PHEOs. These syndromes included multiple endocrine neoplasia type 2A (MEN2A) (found in 42.6% of patients), von Hippel-Lindau Disease (VHL) (found in 19.1% of patients), MEN2B (found in 9.6% of patients), and neurofibromatosis type 1 (found in 8.5% of patients).[8] In another retrospective series that spanned nearly 50 years, 15 of 49 patients (30%) who presented with a unilateral PHEO and had unilateral total adrenalectomy developed a PHEO in the contralateral adrenal gland. This occurred at a median period of 8.2 years after a patient's initial diagnosis (range, 1–20 y).[9] Of the 15 patients who developed PHEOs in the contralateral adrenal gland, 8 had MEN2A, 2 had MEN2B, 2 had VHL, and 1 had a familial PHEO. The risk of developing a contralateral PHEO increased over time. Twenty-five percent of patients developed PHEOs after a median period of 6 years, and 43% of patients developed PHEOs after a median period of 32 years.
PGLs and PHEOs are typically slow-growing tumors, and some may be present for many years before coming to clinical attention. A minority of these tumors are malignant and present with an aggressive clinical course. PGL and PHEO malignancy is defined by the presence of metastases at sites distant from the primary tumor in nonchromaffin tissue. Common sites of metastases include the bone, liver, and lungs.[1,10,11]
There are a lack of reliable molecular, immunohistochemical, and genetic predictors that distinguish between benign and malignant tumors.[12] However, in some studies, multivariate analysis indicates that certain factors lead to a higher malignancy rate (up to 70% in one study).[13] These factors include the following: an SDHB pathogenic variant,[14,15] young patient age,[13,15] extra-adrenal tumors,[15] and large tumors.[16] Some experts view local invasion into surrounding tissue as an additional marker of malignancy.[11,17,18] Others have disagreed with this classification because locally invasive tumors tend to follow a more indolent course than tumors with distant metastatic involvement.[19-23] Consequently, it is difficult to estimate the rate of malignancy in patients with PGLs. Studies have reported malignancy rates that range from 5% to 20%. Certain gene-specific malignancy estimates may be higher or lower than these percentages.[24]
Clinical Diagnosis of PHEO and PGL
A PGL may cause a variety of symptoms depending on the location of the tumor and whether the tumor has secretory capacity. PGLs of the head and neck are rarely associated with elevated catecholamines. Secretory PGLs and PHEOs may cause hypertension, headache, tachycardia, sweating, and flushing. Typically, nonsecretory tumors are painless, coming to attention only when growth of the lesion into surrounding structures causes a mass effect. Patients with a head or neck PGL may present with an enlarging lateral neck mass, hoarseness, Horner syndrome, pulsatile tinnitus, dizziness, facial droop, or blurred vision.[25]
Patients with clinically apparent catecholamine excess generally undergo biochemical testing to evaluate the secretory capacity of the tumor(s).[26] This evaluation is best performed by measuring urine and/or plasma fractionated metanephrines (normetanephrine and metanephrine), which yields a higher sensitivity and specificity than directly measuring catecholamines (norepinephrine, dopamine, and epinephrine).[27] For patients whose plasma metanephrines levels are measured, blood is collected after an intravenous catheter has been inserted and the patient has been in a supine position for 15 to 20 minutes.[28] Additionally, the patient should not have food or caffeinated beverages, smoke cigarettes, or engage in strenuous physical activity in the 8 to 12 hours before the blood draw.[28]
Imaging of PGLs is the mainstay of diagnosis; the initial evaluation includes computed tomography (CT) of the neck and chest. Magnetic resonance imaging (MRI) also has utility for the head and neck.[27] PGLs typically appear homogeneous with intense enhancement after administration of intravenous contrast. MRI may also be used to distinguish the tumor from adjacent vascular and skeletal structures. On T2-weighted images, a tumor that is larger than 2 cm is likely to display a classic "salt and pepper" appearance, a reflection of scattered areas of signal void mingled with areas of high signal intensity from increased vascularity.[29] Imaging of PHEOs usually consists of a dedicated CT of the adrenal glands.[30,31]
Nuclear imaging, particularly somatostatin receptor scintigraphy (SRS) in combination with anatomic imaging, may be useful for localization and determination of the extent of disease (multifocality vs. distant metastatic deposits).[32] Benign tumors are reported to be more sensitive to SRS than iodine I 123-metaiodobenzylguanidine (123I-MIBG) imaging. Sensitivity is highest for the head and neck region compared with abdomen PGLs or PHEOs (91% vs. 40% and 42%, respectively).[33] SRS has been reported to be superior to MIBG in detecting metastatic tumors (95% vs. 23%, respectively).[33] 123I-MIBG, however, is highly sensitive for PHEO [33] and positron emission tomography–computed tomography (PET-CT) is very specific for PGLs. Functional imaging for PGLs and/or PHEOs with fluorine F 18-dihydroxyphenylalanine (18F-DOPA), 18F-fluorodopamine, or PET-CT may be particularly helpful in localizing head and neck tumors. Data suggest that the selection of PET tracer used for tumor localization should be centered on the patient’s genetic status, on the basis of the metabolic activity of the various tumors.[14] It has been suggested that patients with SDHx and VHL pathogenic variants are more likely to have higher 18F-fludeoxyglucose activity, which is related to gene activation in response to hypoxia.[14,34] Some SDHB tumors only weakly concentrate 18F-DOPA, and patients with SDHx pathogenic variants may have false-negative results with such scans. Tumors with VHL pathogenic variants may be missed with MIBG scans.[14]
While further study is needed to determine the optimal imaging strategy for each PHEO/PGL case, gallium Ga 68-DOTATATE (68Ga-DOTATATE) PET-CT is a more recently developed, highly sensitive imaging modality that can identify PHEOs/PGLs.[11,35,36] Two small case series found that 68Ga-DOTATATE PET-CT improved PHEO/PGL identification when compared with conventional imaging done with MRI or CT.[37,38] A meta-analysis found that 68Ga-DOTATATE PET-CT exhibited superior performance for PHEO/PGL detection over other functional imaging modalities in patients with PHEOs/PGLs.[36] The pooled detection rate for 68Ga-DOTATATE PET-CT was 93% when compared with 80% for fluorine F 18-fluorohydroxyphenylalanine (18F-FDOPA) PET-CT, 74% for fluorine F 18-fludeoxyglucose (18F-FDG) PET-CT, and 38% for 123I-MIBG scans. However, a recent study of 14 patients examined the performance of 68Ga-DOTATATE PET-CT, 18F-FDG PET-CT, 18F-FDOPA PET-CT, and MRI in visualizing sporadic primary PHEOs. This study found that 18F-FDOPA PET-CT identified 100% of sporadic PHEOs. Further study is needed to determine if a PHEO's genetic status and secretory capacity can drive decision making regarding preferred PHEO imaging modalities.
Genetics, Inheritance, and Genetic Testing for Familial PHEO and PGL Syndrome
A significant proportion of individuals presenting with apparently sporadic PHEO or PGL are carriers of germline pathogenic variants. Up to 33% of patients with apparently sporadic PHEO, and up to 40% of patients with apparently sporadic PGLs, actually have a recognizable germline pathogenic variant in one of the classical PGL/PHEO susceptibility genes.[21,39-44] One study found that in individuals with a single tumor and a negative family history, the likelihood of an inherited pathogenic variant was 11.6%,[21] whereas other groups detected pathogenic variants in 41% of such patients.[43,45] A large population study reported that up to 85% of patients younger than 21 years with a PGL/PHEO had a pathogenic variant. Specifically, 50% of these patients had an SDHB pathogenic variant.[13,46] For more information, see Childhood Pheochromocytoma and Paraganglioma Treatment. Even among carriers of SDHB pathogenic variants, there can be reduced penetrance and delayed onset of disease, which may further obscure the hereditary nature of the disease.[47] Genetic testing is recommended for all patients with PHEOs or PGLs, even in those who have a single PHEO/PGL but do not have personal or family histories of these tumors. Genetic testing is recommended in this scenario because of the high frequency of pathogenic variants associated with PHEO/PGL predisposition syndromes.[27,48]
PGLs and PHEOs can be seen as part of several well-described tumor susceptibility syndromes including von Hippel-Lindau disease (VHL), MEN2, neurofibromatosis type 1, Carney-Stratakis syndrome, and familial paraganglioma (FPGL) syndrome. FPGL is most commonly caused by pathogenic variants in one of the following four genes: SDHA, SDHB, SDHC, and SDHD (collectively referred to as SDHx). The SDHx proteins form part of the succinate dehydrogenase (SDH) complex, which is located on the inner mitochondrial membrane and plays a critical role in cellular energy metabolism.[49] Pathogenic variants in SDHB are most common, followed by SDHD and rarely SDHC and SDHA. Pathogenic variants in the SDHAF2 (also called SDH5), TMEM127, and MAX genes have been described in FPGL/PHEO,[50-53] but these variants are less common. The mechanism of tumor formation has remained elusive. One study suggests that SDHx-associated tumors display a hypermethylator phenotype that is associated with downregulation of important genes involved in the differentiation of neuroendocrine tissues.[54]
The inheritance pattern of FPGL depends on the gene involved. While most families show traditional autosomal dominant inheritance, those with pathogenic variants in SDHAF2 and SDHD show almost exclusive paternal transmission of the phenotype. FPGL/PHEO syndromes are among the rare inherited diseases in which genomic imprinting contributes to the risk of disease. For example, the SDHD pathogenic variant is normally not activated when inherited from the mother, and the risk of FPGL/PHEO syndromes is not increased. There are reports of disease in individuals with maternally inherited SDHD pathogenic variants,[55-58] although the apparent risk is still unknown but appears to be quite low. However, when a pathogenic variant is inherited from the father, the risk for FPGL/PHEO is greatly increased.[59,60] In other words, while the pathogenic variant can be passed down from mother or father, tumors are almost exclusively seen in individuals with paternally inherited pathogenic variants.[59,60] Potential mechanism(s) of tumorigenesis in individuals with maternally inherited pathogenic variants have been described.[58,61] In cases of FPGL not caused by SDHD or SDHAF2 pathogenic variants, first-degree relatives (FDRs) of an affected individual have a 50% chance of carrying the pathogenic variant and are at increased risk of developing PGLs. Because the family history can appear negative in families with lower penetrance pathogenic variants, it is important to offer genetic testing to all unaffected FDRs once the pathogenic variant in the family has been identified.
Genetic testing for hereditary PHEO and PGL syndromes has until recently been largely based on published algorithms,[27] whereby testing is performed stepwise on the basis of factors such as tumor type and location, age at diagnosis, family history, and presence of malignancy.[21,62,63] This approach allowed for cost-effective, targeted testing on the basis of clinical features. Within the last several years, however, next-generation sequencing (NGS) technology has led to a dramatic decrease in the cost and increase in the efficiency of genetic testing, and interrogation of pathogenic variants in 10 to 30 genes for the same cost of testing two or three genes is now possible.[64] These tests are particularly effective for individuals and families who have an atypical presentation or overlapping clinical features where the "best fit" candidate gene is not obvious.[65] Screening through a multigene panel moderately increases the detection rate. In a small series of 87 patients with PHEO, 25.3% of individuals (22 of 87) were found to have germline pathogenic variants on a screening panel that included ten PGL/PHEO-associated genes; 11.7% had germline pathogenic variants in VHL, 6.8% in RET, 2.3% in SDHD, 2.3% in MAX, 1.1% in SDHB, and 1.1% in TMEM127.[66] Apparently sporadic tumors were present in 74.7% of patients (65 of 87).
Genotype-Phenotype Correlations
In FPGL/PHEO, the type and location of tumors, age at onset, and lifetime penetrance vary depending on which genetic variant an individual has. While these correlations can help guide genetic testing and screening decisions, caution must be used since there is a high degree of variability in these conditions. For more information, see Table 5.
Gene | Risk of PGL/PHEO | Primary Location | Risk of Metastatic or Recurrent Disease | Other Associated Features |
---|---|---|---|---|
CNS = central nervous system; FPGL = familial paraganglioma; GIST = gastrointestinal stromal tumor; HNPGL = head and neck paraganglioma; MTC = medullary thyroid cancer; NETs = neuroendocrine tumors; PGL = paraganglioma; PHEO = pheochromocytoma; PHPT = primary hyperparathyroidism; RCC = renal cell carcinoma. | ||||
aAdapted from Fishbein et al.[11] | ||||
NF1 | Up to 13% | PHEO (rare case reports of PGL) | ~12% | Neurofibromas, Lisch nodules, café au lait spots, optic gliomas, skeletal dysplasia |
VHL | 20% | PHEO (bilateral) (rare case reports of PGL) | <5% | RCC (clear cell type), pancreatic NETs, CNS hemangioblastomas (including the retina) |
RET | 50% | PHEO (bilateral) (rare case reports of PGL) | <5% | MTC, PHPT |
SDHA | 10% | PGL, PHEO | 12% | RCC (clear cell type), GIST |
SDHB | 25% | PGL, HNPGL, PHEO | 25%–50% | |
SDHC | Low | HNPGL (unifocal), thoracic PGL | <5% | |
SDHD | 45% | HNPGL (multifocal), PGL, PHEO | <5%–8% | |
SDHAF2 | Low | HNPGL (multifocal) | Low | |
TMEM127 | Low | PHEO, PGL less common | <5% | RCC |
MAX | Unknown | PHEO | Unclear | |
FH | Very Low | PGL | May be high | RCC (papillary type), cutaneous leiomyomas, uterine fibroids |
SDHD pathogenic variants are mainly associated with an increased risk of parasympathetic PGLs. These are more commonly multifocal and located in the head and neck, with a low rate of malignancy.[13,24,67,68] Multiple series showed a risk of 71% for a head and neck tumor in SDHD carriers.[23,69] The lifetime risk for any PGL in any location in SDHD carriers was estimated to be as high as 77% by age 50 years in one series [69] and 90% by age 70 years in a second series.[68] A review of more than 1,700 cases reported in the literature provided similar estimates, suggesting a lifetime penetrance of 86%.[70] In another study of 160 probands and nonprobands with SDHD pathogenic variants, the risk to age 60 years for all PGL/PHEO was 79%, but the risk for nonprobands only to age 60 years was 50%. In this same study, there was a statistically significant higher penetrance for symptomatic tumors associated with SDHD pathogenic variants compared with SDHB.[67]
The SDHD pathogenic variant p.Pro81Leu (P81L) is common, especially among individuals of European ancestry, and has been previously described as having a distinct phenotype with a low risk for PHEO and sympathetic PGL, and almost exclusive presentation of head and neck PGL.[68] In a 2018 study, the risk to age 60 years for PHEO and sympathetic PGL was lower than 5% among probands and nonprobands with P81L and higher than 25% among those with other SDHD pathogenic variants (P = .01).[67]
Pathogenic variants in the SDHB gene are associated with sympathetic PGLs, although PHEO and parasympathetic PGLs also have been described. SDHB PGLs are more commonly located in the abdomen and mediastinum than in the head and neck. While older studies reported a high age-related penetrance,[70] newer data suggest that the penetrance ranges from 9% to 35% by age 50 years.[47,71-74] There is some evidence that the penetrance in SDHB carriers may be lower in females than in males.[67,75]
Symptoms of hormonal hypersecretion in those with pediatric-onset PGL/PHEO were common in one series of pediatric carriers of SDHB germline pathogenic variants; hypertension was seen in 76%, followed by headache in 68%, sweating/diaphoresis in 51%, palpitations in 40%, nausea and/or vomiting in 31%, and flushing in 25%.[13] The rate of malignancy is higher with SDHB than with the other SDH genes, with up to one-third of patients having malignant tumors in most series.[68,69] However, in one study among nonprobands only, the rate of malignant disease to age 60 was only 4.2 %.[67] One single-center study of pediatric SDHB carriers found that 70% of childhood-onset PGL/PHEO patients developed metastatic disease, with a median interval between diagnoses of primary tumors of 4 years (range, 0–26 y).[13] Pathogenic variants in SDHB have also been associated with several other tumors and malignancies, including gastrointestinal stromal tumors (GISTs), pituitary tumors, renal cell carcinoma (RCC), and papillary thyroid cancer.[67-69]
SDHC pathogenic variants are rare, accounting for an estimated 0.5% of all PGLs.[70] In one series of 153 patients with multiple PGLs or a single PGL diagnosed before age 40 years, 3 (2%) had an SDHC pathogenic variant.[40] Another series of 121 index cases from a head and neck PGL registry showed a pathogenic variant rate of 4% (5 of 121), [76] and another series identified 26 SDHC variants among 391 probands with PGL/PHEO (6.6%).[67] SDHC pathogenic variants most commonly cause head and neck PGLs but have been seen in a small number of patients with abdominal PGLs.[21,67,77] Given small populations sizes, lifetime risk estimates for PGL/PHEO associated with pathogenic SDHC variants are limited, but one study of 43 probands and nonprobands found a lifetime risk for all PGL/PHEO to age 60 years of approximately 75%; among nonprobands only, the risk was 25% (95% confidence interval, 0%–57%).[67] Pathogenic variants in SDHB, SDHC, and SDHD can also cause Carney-Stratakis syndrome, which is characterized by the classic dyad of PGLs and GISTs but can also include pituitary and thyroid tumors.[67,78]
Pathogenic variants in SDHA, SDHAF2, MAX, and TMEM127 have also been described; collectively, they account for up to 6% of cases without pathogenic variants in the classical PGL/PHEO genes, with about one-half of these in SDHA.[65]
Although biallelic pathogenic variants in SDHA have long been known to cause the autosomal recessive condition inherited juvenile encephalopathy/Leigh syndrome,[79] it was not until recently that monoallelic pathogenic variants were linked to an increased risk of developing PGL. One series showed a 7.6% incidence of SDHA pathogenic variants in a cohort of 393 patients with PGL in the Netherlands.[80] Tumors most commonly develop in the head and neck, followed by the adrenal glands and abdomen (extra-adrenal).[81,82] In the same series from the Netherlands,[80] the estimated penetrance for non-index pathogenic variant carriers was 10% by age 70 years.
Initially, pathogenic variants in SDHAF2 were described only in head and neck PGLs.[53]
The MAX gene was first described as a PHEO susceptibility gene in 2011 through exome sequencing of three unrelated cases.[50] Three different germline pathogenic variants were identified, and a follow-up series of 59 cases by the same group identified an additional five variants. The MAX protein plays a key role in the development and progression of neural crest cell tumors.[83]
The TMEM127 gene is located on chromosome 2q11.2. It encodes a transmembrane protein that is a negative regulator of mTOR, which regulates multiple cellular processes. A review of 23 patients with TMEM127 pathogenic variants showed that 96% (22 of 23) had a PHEO, and 9% (2 of 23) had a PGL.[70] TMEM127-associated tumors were diagnosed at an average age of 45 years, which is older than the average age of diagnosis in other hereditary PGL/PHEO syndromes. However, individuals with TMEM127 pathogenic variants have a high chance to develop multiple tumors, either synchronously or metachronously. One large study suggested that TMEM127-associated tumors metastasized infrequently; however, the follow-up period was short. RCC occurred frequently in this population. Hence, RCC risk should be considered when creating surveillance plans for patients with TMEM127 pathogenic variants.[84]
Another study looked at TMEM127 and other genes. Here, an additional 58 patients from the European-American-Asian Pheochromocytoma-Paraganglioma Registry Study Group more than doubled the number of previously reported carriers of rare PGL/PHEO predisposition genes SDHA (n = 29), SDHAF2 (n = 1), MAX (n = 8), and TMEM127 (n = 20).[65] The study identified malignant disease in 12% of SDHA pathogenic variant carriers and 10% of TMEM127 carriers, which is significantly higher than previous estimates. Extra-adrenal tumors were common in the cohort (48%), particularly in SDHA carriers (79%) who had an overrepresentation of head and neck tumors (44%). However, no GIST tumors were identified in SDHA carriers in this cohort, compared with frequent reports in previously identified cohorts. SDHA-related tumors occurred in patients as young as 8 years. Tumors associated with MAX pathogenic variants were almost all in the adrenal glands, and frequently bilateral. Overall, penetrance of developing a PGL/PHEO by age 40 years was estimated to be 73% for MAX pathogenic variant carriers, 41% for TMEM127 carriers, and 39% for SDHA carriers. Penetrance was also calculated for pathogenic variant–positive relatives and was significantly lower for these individuals (13%) compared with index patients for SDHA carriers, but not significantly different for MAX or TMEM127 probands and nonprobands. It is important to remember that these relatively small studies are prone to selection and ascertainment biases, as mentioned above. For example, only 22% of family members from this cohort had cascade screening, which affects penetrance calculations. Additionally, the high rates of metastatic disease could represent ascertainment bias of a tertiary care center, and the lack of GIST tumors could be because this was a PGL/PHEO-specific registry, and therefore might not capture the full spectrum of related tumors.[85]
Surveillance
Patients with an identified germline pathogenic variant in one of the SDH genes are at a significantly increased risk of developing PGLs, PHEOs, renal tumors, and GISTs. PHEOs and PGLs typically have a slow growth pattern, but unchecked growth can lead to mass effect and, ultimately, neurologic compromise. Further, although most of these tumors are benign, some may undergo malignant transformation. As such, periodic screening for interval development of a tumor is of critical importance because early detection and removal can minimize risk to the patient.[15] Although limited studies have been performed to delineate the ideal protocol, total-body MRI has been proposed as a reasonable method for screening because of its high sensitivity and minimal radiation exposure.[27,86] In one study, 37 carriers of SDHx pathogenic variants underwent annual biochemical testing and annual or biennial whole-body MRI beginning at age 10 years.[87] This screening protocol identified six tumors in five patients. The sensitivity of MRI was 87.5%, and the specificity was 94.7%. The sensitivity of biochemical testing was significantly lower at 37.5%, with a specificity similar to MRI at 94.9%.[87] A retrospective study of 157 patients evaluated a rapid contrast-enhanced angio-MRI protocol for the detection of head and neck paragangliomas in carriers of SDH pathogenic variants.[88] This protocol had a high sensitivity and specificity of 88.7% and 93.7%, respectively. Another group, analyzing their experience with a cohort of 157 patients, proposed an algorithm of sequential queries into tumor characteristics to identify those at greatest risk for malignant tumors, and therefore more rigorous surveillance. The presence of any one of the following features including extra-adrenal location, positive family history, positive genetic testing, or tumor size greater than 4 cm was associated with a 100% sensitivity and a 42.5% specificity for the identification of malignancy. The authors further suggested that long-term surveillance may be de-escalated for those patients with small adrenal tumors (4 cm or smaller), as none developed a malignancy in their cohort after a mean follow-up of 7.3 years.[15] Prospective validation of these findings at another institution would be valuable.
Although the optimal imaging protocol in SDH pathogenic variant carriers remains unclear, annual biochemical testing and clinical surveillance may be considered. A combined approach of imaging and biochemical testing may be beneficial, since biochemical testing can miss up to 29% of SDH-related tumors, and imaging has varying sensitivity rates based on the radioisotope that is used.[89] Biochemical testing can be performed by measuring plasma-free metanephrines/catecholamines or 24-hour urinary excretion of fractionated catecholamines (including methoxytyramine, a dopamine metabolite, if available). Clinical surveillance may include physical examination and blood pressure measurement. Clinical surveillance and biochemical testing may begin between ages 5 years and 10 years, or 10 years earlier than the earliest age at diagnosis in the family.[90,91] It is unknown whether continued surveillance beyond age 50 to 60 years is beneficial when a patient is asymptomatic. This is an active area of investigation.[92] One highly specialized center has recommended beginning surveillance at age 6 years for SDHB carriers.[13]
Interventions
Preoperative management
Medical management is the bridge to surgical resection of PGLs/PHEOs. Preoperative medical therapy is not essential for patients without evidence of catecholamine hypersecretion, although some advocate its use regardless of the results of hormonal testing.[28] The aim of pharmacologic therapy is to control hypertension for at least 10 to 14 days before surgery.[93] Management is aimed at preventing catecholamine-induced complications, even in patients who may not present with preoperative hypertension, to avoid intraoperative hypertensive crisis, cardiac arrhythmias, pulmonary edema, and cardiac ischemia. Failure to adequately block the catecholamine excess can dramatically increase the risk of perioperative mortality from hypertensive crisis and lethal arrhythmias and cause hypotensive crisis after tumor removal.[94,95]
In the absence of a randomized controlled trial comparing the various regimens, there is no universally recommended approach. The alpha-adrenoreceptor blocker phenoxybenzamine (Dibenzyline) is most frequently used to control blood pressure and expand the blood volume.[28] Other alpha-blocking drugs have also been used with success, including prazosin, terazosin, or doxazosin; these drugs are more specific alpha-1 adrenergic competitive antagonists and have a shorter half-life than phenoxybenzamine.[96,97] The noncompetitive binding of phenoxybenzamine to the alpha receptors, coupled with its longer half-life, may result in a sustained effect of the drug, with some patients experiencing postoperative hypotension.[28,98] One study found that patients treated with sustained-release doxazosin had more stable perioperative hemodynamic changes and a shorter time interval to preoperative blood pressure control than did patients who received phenoxybenzamine.[98]
A high-volume tertiary care center proposed deviating from the general recommendation for perioperative alpha-receptor blockade. In a closed-case series that compared patients with and without blockade, there was no significant difference in maximal intraoperative systolic arterial pressure or hypertensive episodes.[99] Further study is warranted.
Once the alpha blockade is initiated, expansion of the blood volume is often necessary, as these patients are typically volume contracted.[100,101] In addition to the vasodilatory effects from alpha blockade, volume expansion may be achieved by consuming a high-sodium diet and high fluid intake or a preoperative saline infusion. A clinical manifestation of adequate blockade is the symptom of nasal stuffiness or lightheadedness.
Calcium channel blockers such as nicardipine or nifedipine also have been employed to control the hypertension preoperatively.[102] A calcium channel blocker may be used in conjunction with alpha and beta blockades for refractory hypertension. A calcium channel blocker can also be used alone as a second-line agent for patients with intolerable side effects from the alpha blockade.[28]
Consideration of preoperative imaging is warranted if a pathogenic variant has been identified in a PHEO/PGL syndrome gene. This may alter an individual's surgical plan and approach.[46] This imaging usually includes a dedicated CT scan of the adrenal glands to visualize PHEOs prior to surgery. This scan can help determine if a cortical-sparing adrenalectomy approach is feasible.[30,31] For more information, see the Clinical Diagnosis of PGL and PHEO section.
Surgery
Surgical resection is the treatment of choice for PGL and PHEO. Both open resection and laparoscopic approaches are safe, but if feasible, laparoscopic removal is preferred.[90,103] Open resection is commonly recommended for large tumors (>6 cm–7 cm) because of the increased risk of technical difficulty within the confined space of laparoscopy. Means of exposure and approach are based on the anatomic location of the tumor. Direct access to the adrenal and para-aortic region can be achieved with the posterior approach. It is direct, safe, and efficient.[104] Adequate exposure of the complete tumor is important for complete removal. Robotic assistance can be utilized in select cases because it offers a three-dimensional, magnified view of the anatomy.[105] The efficacy and safety of posterior retroperitoneoscopic adrenalectomy is established, but ongoing studies are examining the relevance of this approach in familial syndromes (for more information, see NCT02618694).
PGLs are commonly located in the para-aortic retroperitoneal sympathetic chain above the aortic bifurcation, below the takeoff of the inferior mesenteric artery (organ of Zuckerkandl), or near the dome of the bladder.[106,107] Malignant PGLs have a dense fibrous capsule that may adhere to surrounding vascularity, which can make complete resection difficult or unfeasible.[11,107] Regional lymph nodes may be involved with malignant tumors, and if suspected preoperatively or noted intraoperatively, a regional lymphadenectomy may be performed.
Genetic testing is best performed before the initial surgery to inform the risk of recurrent or contralateral disease and to guide the extent of resection (e.g., whether to preserve the cortex) because synchronous or metachronous bilateral disease is quite common in hereditary PHEO. Preoperative knowledge of a germline pathogenic variant significantly affects variables associated with a cortical-sparing adrenalectomy. Preserving the cortex is important in patients with a known pathogenic variant because they are at risk of developing a contralateral tumor. Cortical sparing reduces the possibility of future adrenal insufficiency with contralateral adrenalectomy. This consideration must be weighed against the high risk of malignancy in SDHB carriers. Cortical-sparing surgery is an attractive option because it minimizes the risk of adrenal insufficiency and the need for lifelong steroid supplementation. In large series of patients, cortical-sparing surgery has a 3% to 7% recurrence rate after cortical preservation versus a 2% to 3% recurrence rate after total resection (recurrence in the adrenal bed).[9,108] The frequency of steroid dependence in both studies was lower in patients who underwent cortical-sparing techniques than in patients who did not (57% compared with 86%). One of 39 patients (3%) developed adrenal insufficiency after a cortical-sparing procedure; 5 of 25 patients (20%) developed adrenal insufficiency after total adrenalectomy.[9] These study authors recommend cortical-sparing surgery as a viable option for patients with hereditary PHEO, including patients who initially present with seemingly unilateral disease.
References
- Inherited tumour syndromes. In: Lloyd RV, Osamura RY, Klöppel G, et al.: WHO Classification of Tumours of Endocrine Organs. 4th ed. International Agency for Research on Cancer, 2017, pp. 262–66.
- Offergeld C, Brase C, Yaremchuk S, et al.: Head and neck paragangliomas: clinical and molecular genetic classification. Clinics (Sao Paulo) 67 (Suppl 1): 19-28, 2012. [PUBMED Abstract]
- Raygada M, Pasini B, Stratakis CA: Hereditary paragangliomas. Adv Otorhinolaryngol 70: 99-106, 2011. [PUBMED Abstract]
- McNeil AR, Blok BH, Koelmeyer TD, et al.: Phaeochromocytomas discovered during coronial autopsies in Sydney, Melbourne and Auckland. Aust N Z J Med 30 (6): 648-52, 2000. [PUBMED Abstract]
- O'Riordain DS, Young WF, Grant CS, et al.: Clinical spectrum and outcome of functional extraadrenal paraganglioma. World J Surg 20 (7): 916-21; discussion 922, 1996. [PUBMED Abstract]
- Erickson D, Kudva YC, Ebersold MJ, et al.: Benign paragangliomas: clinical presentation and treatment outcomes in 236 patients. J Clin Endocrinol Metab 86 (11): 5210-6, 2001. [PUBMED Abstract]
- Nockel P, El Lakis M, Gaitanidis A, et al.: Preoperative genetic testing in pheochromocytomas and paragangliomas influences the surgical approach and the extent of adrenal surgery. Surgery 163 (1): 191-196, 2018. [PUBMED Abstract]
- Kittah NE, Gruber LM, Bancos I, et al.: Bilateral pheochromocytoma: Clinical characteristics, treatment and longitudinal follow-up. Clin Endocrinol (Oxf) 93 (3): 288-295, 2020. [PUBMED Abstract]
- Grubbs EG, Rich TA, Ng C, et al.: Long-term outcomes of surgical treatment for hereditary pheochromocytoma. J Am Coll Surg 216 (2): 280-9, 2013. [PUBMED Abstract]
- Eisenhofer G, Lenders JW, Timmers H, et al.: Measurements of plasma methoxytyramine, normetanephrine, and metanephrine as discriminators of different hereditary forms of pheochromocytoma. Clin Chem 57 (3): 411-20, 2011. [PUBMED Abstract]
- Fishbein L, Del Rivero J, Else T, et al.: The North American Neuroendocrine Tumor Society Consensus Guidelines for Surveillance and Management of Metastatic and/or Unresectable Pheochromocytoma and Paraganglioma. Pancreas 50 (4): 469-493, 2021. [PUBMED Abstract]
- Jovanovic R, Kostadinova-Kunovska S, Bogoeva B, et al.: Histological features, Ki-67 and Bcl-2 immunohistochemical expression and their correlation with the aggressiveness of pheochromocytomas. Prilozi 33 (2): 23-40, 2012. [PUBMED Abstract]
- Jochmanova I, Abcede AMT, Guerrero RJS, et al.: Clinical characteristics and outcomes of SDHB-related pheochromocytoma and paraganglioma in children and adolescents. J Cancer Res Clin Oncol 146 (4): 1051-1063, 2020. [PUBMED Abstract]
- Taïeb D, Neumann H, Rubello D, et al.: Modern nuclear imaging for paragangliomas: beyond SPECT. J Nucl Med 53 (2): 264-74, 2012. [PUBMED Abstract]
- Dhir M, Li W, Hogg ME, et al.: Clinical Predictors of Malignancy in Patients with Pheochromocytoma and Paraganglioma. Ann Surg Oncol 24 (12): 3624-3630, 2017. [PUBMED Abstract]
- Eisenhofer G, Lenders JW, Siegert G, et al.: Plasma methoxytyramine: a novel biomarker of metastatic pheochromocytoma and paraganglioma in relation to established risk factors of tumour size, location and SDHB mutation status. Eur J Cancer 48 (11): 1739-49, 2012. [PUBMED Abstract]
- Eisenhofer G, Bornstein SR, Brouwers FM, et al.: Malignant pheochromocytoma: current status and initiatives for future progress. Endocr Relat Cancer 11 (3): 423-36, 2004. [PUBMED Abstract]
- Zarnegar R, Kebebew E, Duh QY, et al.: Malignant pheochromocytoma. Surg Oncol Clin N Am 15 (3): 555-71, 2006. [PUBMED Abstract]
- Medeiros LJ, Wolf BC, Balogh K, et al.: Adrenal pheochromocytoma: a clinicopathologic review of 60 cases. Hum Pathol 16 (6): 580-9, 1985. [PUBMED Abstract]
- Walz MK, Alesina PF, Wenger FA, et al.: Laparoscopic and retroperitoneoscopic treatment of pheochromocytomas and retroperitoneal paragangliomas: results of 161 tumors in 126 patients. World J Surg 30 (5): 899-908, 2006. [PUBMED Abstract]
- Mannelli M, Castellano M, Schiavi F, et al.: Clinically guided genetic screening in a large cohort of italian patients with pheochromocytomas and/or functional or nonfunctional paragangliomas. J Clin Endocrinol Metab 94 (5): 1541-7, 2009. [PUBMED Abstract]
- Lee JH, Barich F, Karnell LH, et al.: National Cancer Data Base report on malignant paragangliomas of the head and neck. Cancer 94 (3): 730-7, 2002. [PUBMED Abstract]
- Niemeijer ND, Rijken JA, Eijkelenkamp K, et al.: The phenotype of SDHB germline mutation carriers: a nationwide study. Eur J Endocrinol 177 (2): 115-125, 2017. [PUBMED Abstract]
- Lee H, Jeong S, Yu Y, et al.: Risk of metastatic pheochromocytoma and paraganglioma in SDHx mutation carriers: a systematic review and updated meta-analysis. J Med Genet 57 (4): 217-225, 2020. [PUBMED Abstract]
- DeLellis RA, Lloyd RV, Heitz PU, et al., eds.: Pathology and Genetics of Tumours of Endocrine Organs. IARC Press, 2004. World Health Organization classification of tumours, vol. 8.
- Grossman A, Pacak K, Sawka A, et al.: Biochemical diagnosis and localization of pheochromocytoma: can we reach a consensus? Ann N Y Acad Sci 1073: 332-47, 2006. [PUBMED Abstract]
- Lenders JW, Duh QY, Eisenhofer G, et al.: Pheochromocytoma and paraganglioma: an endocrine society clinical practice guideline. J Clin Endocrinol Metab 99 (6): 1915-42, 2014. [PUBMED Abstract]
- Chen H, Sippel RS, O'Dorisio MS, et al.: The North American Neuroendocrine Tumor Society consensus guideline for the diagnosis and management of neuroendocrine tumors: pheochromocytoma, paraganglioma, and medullary thyroid cancer. Pancreas 39 (6): 775-83, 2010. [PUBMED Abstract]
- Olsen WL, Dillon WP, Kelly WM, et al.: MR imaging of paragangliomas. AJR Am J Roentgenol 148 (1): 201-4, 1987. [PUBMED Abstract]
- Pacak K, Eisenhofer G, Ahlman H, et al.: Pheochromocytoma: recommendations for clinical practice from the First International Symposium. October 2005. Nat Clin Pract Endocrinol Metab 3 (2): 92-102, 2007. [PUBMED Abstract]
- Guerin C, Romanet P, Taieb D, et al.: Looking beyond the thyroid: advances in the understanding of pheochromocytoma and hyperparathyroidism phenotypes in MEN2 and of non-MEN2 familial forms. Endocr Relat Cancer 25 (2): T15-T28, 2018. [PUBMED Abstract]
- Gimenez-Roqueplo AP, Dahia PL, Robledo M: An update on the genetics of paraganglioma, pheochromocytoma, and associated hereditary syndromes. Horm Metab Res 44 (5): 328-33, 2012. [PUBMED Abstract]
- Michałowska I, Ćwikła JB, Pęczkowska M, et al.: Usefulness of Somatostatin Receptor Scintigraphy (Tc-[HYNIC, Tyr3]-Octreotide) and 123I-Metaiodobenzylguanidine Scintigraphy in Patients with SDHx Gene-Related Pheochromocytomas and Paragangliomas Detected by Computed Tomography. Neuroendocrinology 101 (4): 321-30, 2015. [PUBMED Abstract]
- Span PN, Rao JU, Oude Ophuis SB, et al.: Overexpression of the natural antisense hypoxia-inducible factor-1alpha transcript is associated with malignant pheochromocytoma/paraganglioma. Endocr Relat Cancer 18 (3): 323-31, 2011. [PUBMED Abstract]
- Janssen I, Blanchet EM, Adams K, et al.: Superiority of [68Ga]-DOTATATE PET/CT to Other Functional Imaging Modalities in the Localization of SDHB-Associated Metastatic Pheochromocytoma and Paraganglioma. Clin Cancer Res 21 (17): 3888-95, 2015. [PUBMED Abstract]
- Han S, Suh CH, Woo S, et al.: Performance of 68Ga-DOTA-Conjugated Somatostatin Receptor-Targeting Peptide PET in Detection of Pheochromocytoma and Paraganglioma: A Systematic Review and Metaanalysis. J Nucl Med 60 (3): 369-376, 2019. [PUBMED Abstract]
- Kong G, Schenberg T, Yates CJ, et al.: The Role of 68Ga-DOTA-Octreotate PET/CT in Follow-Up of SDH-Associated Pheochromocytoma and Paraganglioma. J Clin Endocrinol Metab 104 (11): 5091-5099, 2019. [PUBMED Abstract]
- Jha A, Ling A, Millo C, et al.: Superiority of 68Ga-DOTATATE over 18F-FDG and anatomic imaging in the detection of succinate dehydrogenase mutation (SDHx )-related pheochromocytoma and paraganglioma in the pediatric population. Eur J Nucl Med Mol Imaging 45 (5): 787-797, 2018. [PUBMED Abstract]
- Jafri M, Whitworth J, Rattenberry E, et al.: Evaluation of SDHB, SDHD and VHL gene susceptibility testing in the assessment of individuals with non-syndromic phaeochromocytoma, paraganglioma and head and neck paraganglioma. Clin Endocrinol (Oxf) 78 (6): 898-906, 2013. [PUBMED Abstract]
- Pęczkowska M, Kowalska A, Sygut J, et al.: Testing new susceptibility genes in the cohort of apparently sporadic phaeochromocytoma/paraganglioma patients with clinical characteristics of hereditary syndromes. Clin Endocrinol (Oxf) 79 (6): 817-23, 2013. [PUBMED Abstract]
- Karasek D, Frysak Z, Pacak K: Genetic testing for pheochromocytoma. Curr Hypertens Rep 12 (6): 456-64, 2010. [PUBMED Abstract]
- Neumann HP, Bausch B, McWhinney SR, et al.: Germ-line mutations in nonsyndromic pheochromocytoma. N Engl J Med 346 (19): 1459-66, 2002. [PUBMED Abstract]
- Fishbein L, Merrill S, Fraker DL, et al.: Inherited mutations in pheochromocytoma and paraganglioma: why all patients should be offered genetic testing. Ann Surg Oncol 20 (5): 1444-50, 2013. [PUBMED Abstract]
- Gómez AM, Soares DC, Costa AAB, et al.: Pheochromocytoma and paraganglioma: implications of germline mutation investigation for treatment, screening, and surveillance. Arch Endocrinol Metab 63 (4): 369-375, 2019. [PUBMED Abstract]
- Bacca A, Sellari Franceschini S, Carrara D, et al.: Sporadic or familial head neck paragangliomas enrolled in a single center: clinical presentation and genotype/phenotype correlations. Head Neck 35 (1): 23-7, 2013. [PUBMED Abstract]
- Babic B, Patel D, Aufforth R, et al.: Pediatric patients with pheochromocytoma and paraganglioma should have routine preoperative genetic testing for common susceptibility genes in addition to imaging to detect extra-adrenal and metastatic tumors. Surgery 161 (1): 220-227, 2017. [PUBMED Abstract]
- Rijken JA, Niemeijer ND, Corssmit EP, et al.: Low penetrance of paraganglioma and pheochromocytoma in an extended kindred with a germline SDHB exon 3 deletion. Clin Genet 89 (1): 128-32, 2016. [PUBMED Abstract]
- Yip L, Duh QY, Wachtel H, et al.: American Association of Endocrine Surgeons Guidelines for Adrenalectomy: Executive Summary. JAMA Surg 157 (10): 870-877, 2022. [PUBMED Abstract]
- Hussain I, Husain Q, Baredes S, et al.: Molecular genetics of paragangliomas of the skull base and head and neck region: implications for medical and surgical management. J Neurosurg 120 (2): 321-30, 2014. [PUBMED Abstract]
- Comino-Méndez I, Gracia-Aznárez FJ, Schiavi F, et al.: Exome sequencing identifies MAX mutations as a cause of hereditary pheochromocytoma. Nat Genet 43 (7): 663-7, 2011. [PUBMED Abstract]
- Neumann HP, Sullivan M, Winter A, et al.: Germline mutations of the TMEM127 gene in patients with paraganglioma of head and neck and extraadrenal abdominal sites. J Clin Endocrinol Metab 96 (8): E1279-82, 2011. [PUBMED Abstract]
- Burnichon N, Lepoutre-Lussey C, Laffaire J, et al.: A novel TMEM127 mutation in a patient with familial bilateral pheochromocytoma. Eur J Endocrinol 164 (1): 141-5, 2011. [PUBMED Abstract]
- Bayley JP, Kunst HP, Cascon A, et al.: SDHAF2 mutations in familial and sporadic paraganglioma and phaeochromocytoma. Lancet Oncol 11 (4): 366-72, 2010. [PUBMED Abstract]
- Letouzé E, Martinelli C, Loriot C, et al.: SDH mutations establish a hypermethylator phenotype in paraganglioma. Cancer Cell 23 (6): 739-52, 2013. [PUBMED Abstract]
- Bayley JP, Oldenburg RA, Nuk J, et al.: Paraganglioma and pheochromocytoma upon maternal transmission of SDHD mutations. BMC Med Genet 15: 111, 2014. [PUBMED Abstract]
- Pigny P, Vincent A, Cardot Bauters C, et al.: Paraganglioma after maternal transmission of a succinate dehydrogenase gene mutation. J Clin Endocrinol Metab 93 (5): 1609-15, 2008. [PUBMED Abstract]
- Burnichon N, Mazzella JM, Drui D, et al.: Risk assessment of maternally inherited SDHD paraganglioma and phaeochromocytoma. J Med Genet 54 (2): 125-133, 2017. [PUBMED Abstract]
- Yeap PM, Tobias ES, Mavraki E, et al.: Molecular analysis of pheochromocytoma after maternal transmission of SDHD mutation elucidates mechanism of parent-of-origin effect. J Clin Endocrinol Metab 96 (12): E2009-13, 2011. [PUBMED Abstract]
- van der Mey AG, Maaswinkel-Mooy PD, Cornelisse CJ, et al.: Genomic imprinting in hereditary glomus tumours: evidence for new genetic theory. Lancet 2 (8675): 1291-4, 1989. [PUBMED Abstract]
- Baysal BE: Mitochondrial complex II and genomic imprinting in inheritance of paraganglioma tumors. Biochim Biophys Acta 1827 (5): 573-7, 2013. [PUBMED Abstract]
- Hensen EF, Jordanova ES, van Minderhout IJ, et al.: Somatic loss of maternal chromosome 11 causes parent-of-origin-dependent inheritance in SDHD-linked paraganglioma and phaeochromocytoma families. Oncogene 23 (23): 4076-83, 2004. [PUBMED Abstract]
- Amar L, Bertherat J, Baudin E, et al.: Genetic testing in pheochromocytoma or functional paraganglioma. J Clin Oncol 23 (34): 8812-8, 2005. [PUBMED Abstract]
- Neumann HP, Erlic Z, Boedeker CC, et al.: Clinical predictors for germline mutations in head and neck paraganglioma patients: cost reduction strategy in genetic diagnostic process as fall-out. Cancer Res 69 (8): 3650-6, 2009. [PUBMED Abstract]
- Toledo RA, Burnichon N, Cascon A, et al.: Consensus Statement on next-generation-sequencing-based diagnostic testing of hereditary phaeochromocytomas and paragangliomas. Nat Rev Endocrinol 13 (4): 233-247, 2017. [PUBMED Abstract]
- Bausch B, Schiavi F, Ni Y, et al.: Clinical Characterization of the Pheochromocytoma and Paraganglioma Susceptibility Genes SDHA, TMEM127, MAX, and SDHAF2 for Gene-Informed Prevention. JAMA Oncol 3 (9): 1204-1212, 2017. [PUBMED Abstract]
- Sbardella E, Cranston T, Isidori AM, et al.: Routine genetic screening with a multi-gene panel in patients with pheochromocytomas. Endocrine 59 (1): 175-182, 2018. [PUBMED Abstract]
- Andrews KA, Ascher DB, Pires DEV, et al.: Tumour risks and genotype-phenotype correlations associated with germline variants in succinate dehydrogenase subunit genes SDHB, SDHC and SDHD. J Med Genet 55 (6): 384-394, 2018. [PUBMED Abstract]
- Ricketts CJ, Forman JR, Rattenberry E, et al.: Tumor risks and genotype-phenotype-proteotype analysis in 358 patients with germline mutations in SDHB and SDHD. Hum Mutat 31 (1): 41-51, 2010. [PUBMED Abstract]
- Neumann HP, Pawlu C, Peczkowska M, et al.: Distinct clinical features of paraganglioma syndromes associated with SDHB and SDHD gene mutations. JAMA 292 (8): 943-51, 2004. [PUBMED Abstract]
- Welander J, Söderkvist P, Gimm O: Genetics and clinical characteristics of hereditary pheochromocytomas and paragangliomas. Endocr Relat Cancer 18 (6): R253-76, 2011. [PUBMED Abstract]
- Schiavi F, Milne RL, Anda E, et al.: Are we overestimating the penetrance of mutations in SDHB? Hum Mutat 31 (6): 761-2, 2010. [PUBMED Abstract]
- Solis DC, Burnichon N, Timmers HJ, et al.: Penetrance and clinical consequences of a gross SDHB deletion in a large family. Clin Genet 75 (4): 354-63, 2009. [PUBMED Abstract]
- Hes FJ, Weiss MM, Woortman SA, et al.: Low penetrance of a SDHB mutation in a large Dutch paraganglioma family. BMC Med Genet 11: 92, 2010. [PUBMED Abstract]
- Rijken JA, Niemeijer ND, Jonker MA, et al.: The penetrance of paraganglioma and pheochromocytoma in SDHB germline mutation carriers. Clin Genet 93 (1): 60-66, 2018. [PUBMED Abstract]
- Jochmanova I, Wolf KI, King KS, et al.: SDHB-related pheochromocytoma and paraganglioma penetrance and genotype-phenotype correlations. J Cancer Res Clin Oncol 143 (8): 1421-1435, 2017. [PUBMED Abstract]
- Schiavi F, Boedeker CC, Bausch B, et al.: Predictors and prevalence of paraganglioma syndrome associated with mutations of the SDHC gene. JAMA 294 (16): 2057-63, 2005. [PUBMED Abstract]
- Peczkowska M, Cascon A, Prejbisz A, et al.: Extra-adrenal and adrenal pheochromocytomas associated with a germline SDHC mutation. Nat Clin Pract Endocrinol Metab 4 (2): 111-5, 2008. [PUBMED Abstract]
- Pasini B, McWhinney SR, Bei T, et al.: Clinical and molecular genetics of patients with the Carney-Stratakis syndrome and germline mutations of the genes coding for the succinate dehydrogenase subunits SDHB, SDHC, and SDHD. Eur J Hum Genet 16 (1): 79-88, 2008. [PUBMED Abstract]
- Horváth R, Abicht A, Holinski-Feder E, et al.: Leigh syndrome caused by mutations in the flavoprotein (Fp) subunit of succinate dehydrogenase (SDHA). J Neurol Neurosurg Psychiatry 77 (1): 74-6, 2006. [PUBMED Abstract]
- van der Tuin K, Mensenkamp AR, Tops CMJ, et al.: Clinical Aspects of SDHA-Related Pheochromocytoma and Paraganglioma: A Nationwide Study. J Clin Endocrinol Metab 103 (2): 438-445, 2018. [PUBMED Abstract]
- Burnichon N, Brière JJ, Libé R, et al.: SDHA is a tumor suppressor gene causing paraganglioma. Hum Mol Genet 19 (15): 3011-20, 2010. [PUBMED Abstract]
- Korpershoek E, Favier J, Gaal J, et al.: SDHA immunohistochemistry detects germline SDHA gene mutations in apparently sporadic paragangliomas and pheochromocytomas. J Clin Endocrinol Metab 96 (9): E1472-6, 2011. [PUBMED Abstract]
- Grandori C, Cowley SM, James LP, et al.: The Myc/Max/Mad network and the transcriptional control of cell behavior. Annu Rev Cell Dev Biol 16: 653-99, 2000. [PUBMED Abstract]
- Armaiz-Pena G, Flores SK, Cheng ZM, et al.: Genotype-Phenotype Features of Germline Variants of the TMEM127 Pheochromocytoma Susceptibility Gene: A 10-Year Update. J Clin Endocrinol Metab 106 (1): e350-e364, 2021. [PUBMED Abstract]
- Fishbein L, Nathanson KL: Pheochromocytoma and Paraganglioma Susceptibility Genes: Estimating the Associated Risk of Disease. JAMA Oncol 3 (9): 1212-1213, 2017. [PUBMED Abstract]
- Schiffman JD: No child left behind in SDHB testing for paragangliomas and pheochromocytomas. J Clin Oncol 29 (31): 4070-2, 2011. [PUBMED Abstract]
- Jasperson KW, Kohlmann W, Gammon A, et al.: Role of rapid sequence whole-body MRI screening in SDH-associated hereditary paraganglioma families. Fam Cancer 13 (2): 257-65, 2014. [PUBMED Abstract]
- Gravel G, Niccoli P, Rohmer V, et al.: The value of a rapid contrast-enhanced angio-MRI protocol in the detection of head and neck paragangliomas in SDHx mutations carriers: a retrospective study on behalf of the PGL.EVA investigators. Eur Radiol 26 (6): 1696-704, 2016. [PUBMED Abstract]
- Greenberg SE, Jacobs MF, Wachtel H, et al.: Tumor detection rates in screening of individuals with SDHx-related hereditary paraganglioma-pheochromocytoma syndrome. Genet Med 22 (12): 2101-2107, 2020. [PUBMED Abstract]
- Timmers HJ, Gimenez-Roqueplo AP, Mannelli M, et al.: Clinical aspects of SDHx-related pheochromocytoma and paraganglioma. Endocr Relat Cancer 16 (2): 391-400, 2009. [PUBMED Abstract]
- Lefebvre M, Foulkes WD: Pheochromocytoma and paraganglioma syndromes: genetics and management update. Curr Oncol 21 (1): e8-e17, 2014. [PUBMED Abstract]
- Greenberg SE, Holman R, Kohlmann W, et al.: Paraganglioma and other tumour detection rates in individuals with SDHx pathogenic variants by age of diagnosis and after the age of 50. Clin Endocrinol (Oxf) 95 (3): 447-452, 2021. [PUBMED Abstract]
- Pacak K: Preoperative management of the pheochromocytoma patient. J Clin Endocrinol Metab 92 (11): 4069-79, 2007. [PUBMED Abstract]
- GRAHAM JB: Pheochromocytoma and hypertension; an analysis of 207 cases. Int Abstr Surg 92 (2): 105-21, 1951. [PUBMED Abstract]
- Goldstein RE, O'Neill JA, Holcomb GW, et al.: Clinical experience over 48 years with pheochromocytoma. Ann Surg 229 (6): 755-64; discussion 764-6, 1999. [PUBMED Abstract]
- Miura Y, Yoshinaga K: Doxazosin: a newly developed, selective alpha 1-inhibitor in the management of patients with pheochromocytoma. Am Heart J 116 (6 Pt 2): 1785-9, 1988. [PUBMED Abstract]
- Prys-Roberts C, Farndon JR: Efficacy and safety of doxazosin for perioperative management of patients with pheochromocytoma. World J Surg 26 (8): 1037-42, 2002. [PUBMED Abstract]
- Zhu Y, He HC, Su TW, et al.: Selective α1-adrenoceptor antagonist (controlled release tablets) in preoperative management of pheochromocytoma. Endocrine 38 (2): 254-9, 2010. [PUBMED Abstract]
- Groeben H, Nottebaum BJ, Alesina PF, et al.: Perioperative α-receptor blockade in phaeochromocytoma surgery: an observational case series. Br J Anaesth 118 (2): 182-189, 2017. [PUBMED Abstract]
- Ross EJ, Prichard BN, Kaufman L, et al.: Preoperative and operative management of patients with phaeochromocytoma. Br Med J 1 (5534): 191-8, 1967. [PUBMED Abstract]
- Hack HA: The perioperative management of children with phaeochromocytoma. Paediatr Anaesth 10 (5): 463-76, 2000. [PUBMED Abstract]
- Proye C, Thevenin D, Cecat P, et al.: Exclusive use of calcium channel blockers in preoperative and intraoperative control of pheochromocytomas: hemodynamics and free catecholamine assays in ten consecutive patients. Surgery 106 (6): 1149-54, 1989. [PUBMED Abstract]
- Vargas HI, Kavoussi LR, Bartlett DL, et al.: Laparoscopic adrenalectomy: a new standard of care. Urology 49 (5): 673-8, 1997. [PUBMED Abstract]
- Perrier ND, Kennamer DL, Bao R, et al.: Posterior retroperitoneoscopic adrenalectomy: preferred technique for removal of benign tumors and isolated metastases. Ann Surg 248 (4): 666-74, 2008. [PUBMED Abstract]
- Dickson PV, Jimenez C, Chisholm GB, et al.: Posterior retroperitoneoscopic adrenalectomy: a contemporary American experience. J Am Coll Surg 212 (4): 659-65; discussion 665-7, 2011. [PUBMED Abstract]
- Ober WB: Emil Zuckerkandl and his delightful little organ. Pathol Annu 18 Pt 1: 103-19, 1983. [PUBMED Abstract]
- Mundschenk J, Lehnert H: Malignant pheochromocytoma. Exp Clin Endocrinol Diabetes 106 (5): 373-6, 1998. [PUBMED Abstract]
- Castinetti F, Qi XP, Walz MK, et al.: Outcomes of adrenal-sparing surgery or total adrenalectomy in phaeochromocytoma associated with multiple endocrine neoplasia type 2: an international retrospective population-based study. Lancet Oncol 15 (6): 648-55, 2014. [PUBMED Abstract]
Carney-Stratakis Syndrome
Clinical Description
Carney-Stratakis syndrome (CSS; also known as Carney-Stratakis dyad) was first described in 2002. CSS is distinct from similarly named syndromes, Carney Complex and Carney Triad (for more information, see Table 6). CSS is characterized by an autosomal dominant germline pathogenic variant in the succinate dehydrogenase (SDH) subunit B, C, or D (SDHx) genes that demonstrates incomplete penetrance. Affected individuals develop multifocal, locally aggressive gastrointestinal stromal tumors (GISTs) and multiple neck, intrathoracic, and intra-abdominal paragangliomas (PGLs) at relatively early ages.[1-3] CSS-associated GISTs and PGLs display phenotypes that differ from their sporadically occurring, more-common counterparts; as a result, it is important to understand the unique features of imaging, treatment, and surveillance in patients with CSS.
Syndrome | Inheritance Pattern | Mean Age at Onset (y) | Affected Sex | Associated Lesions | Pathogenic Variants | Tumor Behavior |
---|---|---|---|---|---|---|
AD = autosomal dominant; GIST = gastrointestinal stromal tumor; F = female; M = male. | ||||||
Carney-Stratakis syndrome [1,3,4] | AD | 23 | M, F | Paraganglioma, stomach epithelioid GIST | Germline SDHx pathogenic variants common; no KIT or PDGFRA pathogenic variants | GIST metastasis but protracted course; paraganglioma aggressive |
Carney triad [4-6] | None | <30 | >95% F | Lung chondroma, paraganglioma, stomach epithelioid GIST | No KIT or PDGFRA pathogenic variants; rarely, SDHx pathogenic variants (9.5% in one series) [7] | GIST metastasis but protracted course |
Carney complex [8,9] | AD | 20 | M, F | Lentigines, myxomas, schwannoma, thyroid follicular adenomas or carcinoma, primary pigmented nodular adrenocortical disease, pituitary adenomas | Germline PRKAR1A pathogenic variants | N/A |
Genetics, Inheritance, and Genetic Testing for CSS
The tumorigenesis of CSS-associated GISTs appears to involve succinate dehydrogenase deficiency rather than gain-of-function variants in the KIT gene or the PDGFRA gene, as seen in the vast majority of GISTs.[10] SDH deficiency is also a characteristic finding of pediatric-type GISTs; CSS-associated GISTs display clinical findings similar to these tumors, including young age at onset (median age, 19 y), specificity to the stomach, multifocality, and resistance to imatinib.[3,11-13] Furthermore, tumor size and mitotic rate do not accurately predict metastatic potential or survival, as SDH-deficient GISTs frequently metastasize to regional lymph nodes, the peritoneal cavity, and the liver; however, long-term survival is common.[6,14] For more information about genetic testing for Carney-Stratakis syndrome, see the section on Genetics, Inheritance, and Genetic Testing in the Familial Pheochromocytoma and Paraganglioma Syndrome section.
Surveillance
Although the natural history of CSS is poorly understood, experts recommend that ongoing surveillance include the following: close patient follow-up with annual history that focuses on symptoms of anemia and catecholamine excess, physical exam, biochemical analysis with plasma metanephrine level and chromogranin A to detect recurrent PGLs, and cross-sectional imaging. Although many PGLs do not secrete catecholamines, chromogranin A has been found to be elevated in PGLs and may be a useful marker for tumor recurrence. The appropriate screening imaging modality is unknown at this time, but fluorine F 18-fludeoxyglucose positron emission tomography–computed tomography (18F-FDG PET-CT) is highly sensitive at identifying extra-adrenal PGLs and GISTs. Because of the risks of ionizing radiation exposure from CT, some suggest using MRI for annual surveillance.[15,16]
Interventions
Because multiple primary GISTs and PGLs are common with CSS, preoperative imaging is paramount to accurately identify the extent of disease before surgical planning. Most patients will present having already undergone imaging with CT or magnetic resonance imaging (MRI). Both methods have excellent sensitivity for identifying PGLs, but additional functional imaging is recommended because of the diffuse nature of these tumors. 18F-FDG PET-CT is superior to iodine I 123-metaiodobenzylguanidine at identifying SDHx-associated PGLs and, because of the high metabolic activity of GISTs, has excellent sensitivity in identifying them.[15,17] Thus, in patients with SDHx pathogenic variants, including those with CSS, 18F-FDG PET-CT is the preferred functional imaging modality to optimally detect and stage all GISTs and PGLs.[16] Some evidence suggests that fluorine F 18-fluorohydroxyphenylalanine (18F-FDOPA) PET-CT is superior at identifying the primary PGL, while 18F-FDG PET-CT is superior at identifying metastases.
There are no prospective treatment studies involving patients with CSS; therefore, recommendations are based on limited clinical experience, single case series, and extrapolations from genetically-similar tumors with similar clinical behavior. The mainstay of treatment for CSS-associated GISTs and PGLs is complete surgical resection of the tumor. The timing of the operation correlates with the presentation of the tumor. Surgical resection can be accomplished with laparoscopic or open techniques. For PGLs, vascular reconstruction is uncommon. Although PGLs are commonly present in the paraaortic region, the need for major vascular reconstruction is uncommon. GIST tumors can be resected with wedge resection and primary closure and re-anastomosis. Ensuring negative margins is important, as patients for whom a complete resection is accomplished experience the longest survival.[18] In the rare setting of synchronous disease, combined resection is appropriate if tolerable by the patient. More commonly, tumors develop metachronously, with GISTs arising first; individual resection occurs at the time of diagnosis of each tumor.
A thorough preoperative endoscopy and complete surgical exploration of the stomach are essential, as multiple separate GISTs are frequently encountered. The high frequency of multifocality and the likelihood of tumor recurrence do not justify a prophylactic total gastrectomy because of its substantial associated morbidity. Furthermore, a total gastrectomy is generally only performed when the current disease burden precludes a lesser resection. To this end, gastric wedge resection with gross negative margins is the surgical goal.[19] Sampling of any suspicious nodes at the time of resection is commonly performed. Evidence suggests that locally advanced CSS-associated GISTs demonstrate a rather indolent course;[20] thus, the concern for nodal involvement based on preoperative imaging or abdominal exploration need not deter resection of the primary tumor. While a role for neoadjuvant imatinib in locally advanced adult-type GISTs has been widely described to improve resectability or reduce the burden of resection, it is unlikely to have any effect in locally advanced SDH-deficient GISTs.[21] Evidence suggests that for these tumors, the second-line targeted agents, including sorafenib, sunitinib, dasatinib, and nilotinib, may be beneficial in the adjuvant setting.[22,23] No data support using these agents in the neoadjuvant setting at this time.
Regarding treatment of CSS-associated PGLs, patients are commonly initiated on alpha-blockade preoperatively to minimize perioperative cardiac morbidity and mortality. PGLs typically occur in the para-aortic chain from the urinary bladder and the aortic bifurcation to the superior mediastinum and head and neck. As in the treatment of GISTs, the operative goal is resection of all known disease. Preoperative imaging and intra-operative exploration are essential to achieving this goal. Multiple tumors are common; when disease is present in the bilateral adrenal glands, the surgeon faces the possibility of rendering a patient steroid dependent with a lifelong risk of a fatal Addisonian crisis. In this setting, a surgeon proficient in performing a cortical-sparing adrenalectomy may be consulted.
References
- Carney JA, Stratakis CA: Familial paraganglioma and gastric stromal sarcoma: a new syndrome distinct from the Carney triad. Am J Med Genet 108 (2): 132-9, 2002. [PUBMED Abstract]
- McWhinney SR, Pasini B, Stratakis CA, et al.: Familial gastrointestinal stromal tumors and germ-line mutations. N Engl J Med 357 (10): 1054-6, 2007. [PUBMED Abstract]
- Pasini B, McWhinney SR, Bei T, et al.: Clinical and molecular genetics of patients with the Carney-Stratakis syndrome and germline mutations of the genes coding for the succinate dehydrogenase subunits SDHB, SDHC, and SDHD. Eur J Hum Genet 16 (1): 79-88, 2008. [PUBMED Abstract]
- Gaal J, Stratakis CA, Carney JA, et al.: SDHB immunohistochemistry: a useful tool in the diagnosis of Carney-Stratakis and Carney triad gastrointestinal stromal tumors. Mod Pathol 24 (1): 147-51, 2011. [PUBMED Abstract]
- Agaimy A, Pelz AF, Corless CL, et al.: Epithelioid gastric stromal tumours of the antrum in young females with the Carney triad: a report of three new cases with mutational analysis and comparative genomic hybridization. Oncol Rep 18 (1): 9-15, 2007. [PUBMED Abstract]
- Zhang L, Smyrk TC, Young WF, et al.: Gastric stromal tumors in Carney triad are different clinically, pathologically, and behaviorally from sporadic gastric gastrointestinal stromal tumors: findings in 104 cases. Am J Surg Pathol 34 (1): 53-64, 2010. [PUBMED Abstract]
- Boikos SA, Xekouki P, Fumagalli E, et al.: Carney triad can be (rarely) associated with germline succinate dehydrogenase defects. Eur J Hum Genet 24 (4): 569-73, 2016. [PUBMED Abstract]
- Boikos SA, Stratakis CA: Carney complex: pathology and molecular genetics. Neuroendocrinology 83 (3-4): 189-99, 2006. [PUBMED Abstract]
- Correa R, Salpea P, Stratakis CA: Carney complex: an update. Eur J Endocrinol 173 (4): M85-97, 2015. [PUBMED Abstract]
- Hensen EF, Bayley JP: Recent advances in the genetics of SDH-related paraganglioma and pheochromocytoma. Fam Cancer 10 (2): 355-63, 2011. [PUBMED Abstract]
- Agaram NP, Laquaglia MP, Ustun B, et al.: Molecular characterization of pediatric gastrointestinal stromal tumors. Clin Cancer Res 14 (10): 3204-15, 2008. [PUBMED Abstract]
- Miettinen M, Wang ZF, Sarlomo-Rikala M, et al.: Succinate dehydrogenase-deficient GISTs: a clinicopathologic, immunohistochemical, and molecular genetic study of 66 gastric GISTs with predilection to young age. Am J Surg Pathol 35 (11): 1712-21, 2011. [PUBMED Abstract]
- Sawhney SA, Chapman AD, Carney JA, et al.: Incomplete Carney triad--a review of two cases. QJM 102 (9): 649-53, 2009. [PUBMED Abstract]
- Rege TA, Wagner AJ, Corless CL, et al.: "Pediatric-type" gastrointestinal stromal tumors in adults: distinctive histology predicts genotype and clinical behavior. Am J Surg Pathol 35 (4): 495-504, 2011. [PUBMED Abstract]
- Ayala-Ramirez M, Callender GG, Kupferman ME, et al.: Paraganglioma syndrome type 1 in a patient with Carney-Stratakis syndrome. Nat Rev Endocrinol 6 (2): 110-5, 2010. [PUBMED Abstract]
- Timmers HJ, Kozupa A, Chen CC, et al.: Superiority of fluorodeoxyglucose positron emission tomography to other functional imaging techniques in the evaluation of metastatic SDHB-associated pheochromocytoma and paraganglioma. J Clin Oncol 25 (16): 2262-9, 2007. [PUBMED Abstract]
- Timmers HJ, Chen CC, Carrasquillo JA, et al.: Comparison of 18F-fluoro-L-DOPA, 18F-fluoro-deoxyglucose, and 18F-fluorodopamine PET and 123I-MIBG scintigraphy in the localization of pheochromocytoma and paraganglioma. J Clin Endocrinol Metab 94 (12): 4757-67, 2009. [PUBMED Abstract]
- Abadin SS, Ayala-Ramirez M, Jimenez C, et al.: Impact of surgical resection for subdiaphragmatic paragangliomas. World J Surg 38 (3): 733-41, 2014. [PUBMED Abstract]
- Demetri GD, Benjamin RS, Blanke CD, et al.: NCCN Task Force report: management of patients with gastrointestinal stromal tumor (GIST)--update of the NCCN clinical practice guidelines. J Natl Compr Canc Netw 5 (Suppl 2): S1-29; quiz S30, 2007. [PUBMED Abstract]
- Maki RG, Blay JY, Demetri GD, et al.: Key Issues in the Clinical Management of Gastrointestinal Stromal Tumors: An Expert Discussion. Oncologist 20 (7): 823-30, 2015. [PUBMED Abstract]
- Ganjoo KN, Villalobos VM, Kamaya A, et al.: A multicenter phase II study of pazopanib in patients with advanced gastrointestinal stromal tumors (GIST) following failure of at least imatinib and sunitinib. Ann Oncol 25 (1): 236-40, 2014. [PUBMED Abstract]
- Gill AJ, Chou A, Vilain R, et al.: Immunohistochemistry for SDHB divides gastrointestinal stromal tumors (GISTs) into 2 distinct types. Am J Surg Pathol 34 (5): 636-44, 2010. [PUBMED Abstract]
- Janeway KA, Albritton KH, Van Den Abbeele AD, et al.: Sunitinib treatment in pediatric patients with advanced GIST following failure of imatinib. Pediatr Blood Cancer 52 (7): 767-71, 2009. [PUBMED Abstract]
Familial Nonmedullary Thyroid Cancer
Clinical Description
Papillary and follicular cancers, along with their various histologic subtypes, arise from the follicular cells of the thyroid and are collectively referred to as differentiated thyroid cancer or nonmedullary thyroid cancer (NMTC). Papillary thyroid cancer (PTC) is the most common form of thyroid cancer, comprising over 85% of all cases, and is rapidly increasing in incidence worldwide.[1]
Radiation exposure, particularly during childhood, has been extensively studied as a causative factor in the development of thyroid cancer; however, it accounts for only a small minority of cases.[2,3] One of the strongest risk factors for the development of thyroid cancer is a family history of the disease, in which cases are termed familial nonmedullary thyroid cancer (FNMTC). The exact incidence of FNMTC is difficult to determine because the criteria used to qualify as a heritable condition varies among studies. Criteria that have yet to be universally defined include the number of affected relatives and their relationship (i.e., first-degree relatives, second-degree relatives, etc.), pattern of inheritance, and the presence of coexisting thyroid conditions.
Further confounding the distinction between inherited and sporadic disease is the high prevalence of incidental microcarcinomas, which may be found in 10% to 15% of surgeries or autopsies.[4] Because there are, as of yet, no known gene variants responsible for FNMTC in the majority of families, this high background prevalence of disease poses a challenge in assessing the risk of a thyroid malignancy in other family members. This uncertainty may be especially problematic in equivocal cases of inherited disease; for example, two second-degree relatives with thyroid cancer.
FNMTC may be part of a larger syndrome associated with tumors involving other organs or may represent a stand-alone condition. Table 7 outlines the various hereditary syndromes associated with NMTC.
Genetics, Inheritance, and Genetic Testing for Familial NMTC
The genetics of familial medullary thyroid cancer (FMTC) in the context of multiple endocrine neoplasia type 2 are well established. Genetic factors also clearly contribute to NMTC, as it has one of the highest heritabilities of any cancer site, with a relative risk of fivefold to tenfold for relatives of patients, especially (female) siblings.[5-8] FNMTC, which includes follicular subtypes, primarily papillary, is thought to account for 5% to 10% of all NMTC cases.[5,9,10] Notably, when there are only two individuals affected in a family, there is a 40% to 60% chance that the disease is actually sporadic, whereas when three or more family members are affected there is a 96% chance the disease has an inherited component.[11] With the exception of a few rare genetic syndromes with NMTC as a minor component, the majority of FNMTC is nonsyndromic and the underlying genetic predisposition is unclear. Still, the term familial cancer is somewhat misleading as FNMTC pedigrees demonstrate a definitive mendelian pattern of inheritance which is autosomal dominant with incomplete penetrance and variable expressivity.[5,12-15] However, unlike FMTC, FNMTC is a polygenic disease with no single locus responsible for the majority of cases or easily identifiable phenotype and it is likely modified by multiple low-penetrance alleles and environmental factors.[16]
Ruling out syndromic FNMTC
As there is no clinical genetic testing for nonsyndromic FNMTC, identification of at-risk families must rely on astute clinicians obtaining a thorough clinical examination and detailed personal and family history of any patient presenting with thyroid cancer or disease. Aspects of a history that suggest FNMTC include multiple generations affected, early-onset bilateral/multifocal thyroid tumors (especially in males) with a more aggressive clinical course, and association with benign thyroid pathologies.[17] Detailed work-up is critical in FNMTC as it is ultimately a diagnosis of exclusion in the sense that other familial cancer predisposition syndromes associated with NMTC must first be ruled out, such as Cowden syndrome or familial adenomatous polyposis. These differential diagnoses for FNMTC are outlined in Table 7. Notably, the association of NMTC with McCune-Albright, Peutz-Jeghers, ataxia-telangiectasia, and multiple endocrine neoplasia type 1 syndromes is less established.
Syndrome | Gene | Inheritance | Incidence of Thyroid Cancer (%) | Type of Thyroid Cancer | Extrathyroidal Clinical Features |
---|---|---|---|---|---|
FAP = familial adenomatous polyposis; FTC = follicular thyroid cancer; MNG = multinodular goiter; PTC = papillary thyroid cancer. | |||||
aAdapted from Nose,[18] Sturgeon et al.,[19] and Vriens et al.[17] | |||||
FAP/Gardner syndrome | APC | Autosomal dominant | 2 | PTC (cribriform morular variant) | Gastrointestinal adenomatous polyps; Gardner syndrome also includes desmoid tumors, supernumerary teeth, fibrous dysplasia of skull, osteomas, epidermoid cysts, hypertrophy of retinal epithelium |
Cowden syndrome (PTEN hamartoma syndrome) | PTEN (rarely SDHx, KLLN, AKT1, PIK3CA) | Autosomal dominant | 10–35 | FTC, PTC | Malignant tumors and hamartomas of breast, endometrium, thyroid, kidney, gastrointestinal tract, brain, skin |
Carney complex | PRKAR1α | Autosomal dominant | 11–15 | FTC, PTC | Myxomas of soft tissues, skin and mucosal pigmentation (blue nevi), schwannomas, tumors of adrenal, pituitary and testicle |
Werner syndrome | WRN | Autosomal recessive | 18 | FTC, anaplastic PTC | Premature aging (adult progeria), scleroderma-like skin changes, cataracts, subcutaneous calcifications, muscular atrophy, diabetes |
DICER1 syndrome | DICER1 | Autosomal dominant | Unknown | PTC (and MNG) | Familial pleuropulmonary blastoma; cystic nephroma; ovarian Sertoli-Leydig cell tumors |
McCune-Albright syndrome | GNAS | Mosaic somatic variants | Unknown | FTC | Polyostotic fibrous dysplasia, café-au-lait spots, endocrine hyperfunction of pituitary, adrenal, gonadal tissues |
Peutz-Jeghers syndrome | STK11 (LKB1) | Autosomal dominant | Unknown | Primarily PTC | Hamartomas of small intestine, mucocutaneous hyperpigmentation, Sertoli cell testicular tumors |
Ataxia-telangiectasia | ATM | Autosomal recessive | Unknown | Primarily PTC | Cerebellar ataxia and nystagmus, oculocutaneous telangiectasia, immunodeficiency, lymphoreticular cancers |
Multiple endocrine neoplasia type 1 (MEN1) | MEN1 | Autosomal dominant | Unknown | Primarily PTC | Tumors of parathyroid glands, endocrine gastroenteropancreatic tract, anterior pituitary gland |
Identifying genes and inherited variants associated with nonsyndromic FNMTC
Various methods have been employed to uncover the landscape of genetic variation associated with FNMTC, mainly genome-wide linkage analysis using microsatellite markers evenly distributed across the genome and informative large pedigrees with multiple affected family members. More than 15 genetic loci have been linked to FNMTC, which are summarized in Table 8. The loci that are italicized represent those where the susceptibility gene has been identified; the causal genes at the other loci remain unknown. The first four loci were identified by microsatellite linkage analysis. The remaining loci have been identified by increasingly dense single nucleotide variant (SNV) arrays as well as microRNA arrays and, most recently, next-generation sequencing. Most of these studies have been done on groups of families with pedigrees consistent with FNMTC; however, two of the loci were identified through large, population level SNV array analysis. Notably, several studies have excluded the genes that are most commonly somatically altered in association with sporadic NMTC as having a role in FNMTC, namely BRAF, RET, RET/PTC, MET, MEK1, MEK2, RAS, and NTRK.[20] For more information on linkage analysis and next-generation sequencing, see Cancer Genetics Overview.
Locus | Location | Tumor Type | Sample Sizea | Study Type | Original Cohort Country of Origin | Year | References | |
---|---|---|---|---|---|---|---|---|
FTC = follicular thyroid cancer; MNG = multinodular goiter; miRNA = microRNA; NMTC = nonmedullary thyroid cancer; PRN = papillary renal neoplasia; PTC = papillary thyroid cancer; SNV = single nucleotide variant; WES = whole-exome sequencing. | ||||||||
aCombined across studies. | ||||||||
MNG1 | 14q31 | MNG with PTC | 1 kindred | Microsatellite linkage | Canada | 1997 | [21] | |
18 MNG | ||||||||
2 PTC | ||||||||
TCO | 19p13.2 | PTC with oxyphilia | 1 kindred | Microsatellite linkage | France | 1998 | [22-25] | |
20 families | ||||||||
6 MNG | ||||||||
3 PTC | ||||||||
49 NMTC | ||||||||
fPTC/PRN | 1q21 | PTC with PRN | 1 kindred | Microsatellite linkage | United States | 2000 | [26] | |
5 PTC | ||||||||
2 PRN | ||||||||
NMTC1 | 2q21 | PTC (follicular variant) | 1 kindred, 80 pedigrees | Microsatellite linkage | Tasmania | 2001 | [24,25,27] | |
19 families | ||||||||
49 NMTC | ||||||||
FTEN | 8p23.1-p22 | PTC (classic) | 1 kindred | 10K SNV array | Portugal | 2008 | [28] | |
11 benign | ||||||||
5 NMTC | ||||||||
Unknown | 8q24 | PTC with melanoma | 26 families | 50K SNV array | United States | 2009 | [29] | |
FOXE1 | 9q22.33 | PTC/FTC | 60 families | 300K SNV array | Iceland/Spain/United States | 2009 | [30] | |
197 PTC/FTC | ||||||||
NKX2-1/TITF-1 | 14q13.3 | PTC and MNG | 60 families | 300K SNV array | Iceland/United States/Spain | 2009 | [30] | |
197 PTC/FTC | ||||||||
Unknown | 6q22 | PTC/FTC (classic) | 38 families | 50K SNV array | United States/Italy | 2009 | [31] | |
49 PTC | ||||||||
miR-886-3p | 5q31.2 | PTC | 21 PTC | 3K miRNA array | United States | 2011 | [32] | |
7 FNMTC | ||||||||
10 normal thyroid tissue | ||||||||
miR-20a | 13q31.3 | PTC | 21 PTC | 3K miRNA array | United States | 2011 | [32] | |
7 FNMTC | ||||||||
10 normal thyroid tissue | ||||||||
Telomere-telomerase complex (TERT, TRF1, TFR2, RAP1, TIN2, TPP1, POT1) | 5p15.3 (TERT), etc. | PTC | 47 PTC | 2008 | [33] | |||
SRGAP1 | 12q14 | PTC | 38 families | 250K SNV array | United States/Poland | 2013 | [34] | |
HAPB2 | 10q25-26 | PTC, follicular adenoma | 1 kindred | WES | United States | 2015 | [35] | |
7 PTC | ||||||||
RTFC (c14orf93) | 14q11.2 | PTC | 15 families | WES | China | 2017 | [36] |
Susceptibility loci identified through linkage analyses
MNG1, TCO, fPTC/PRN and NMTC1 are proposed FNMTC susceptibility loci identified in families with multiple affected individuals and are summarized in Table 8. Conflicting evidence exists regarding the linkage to the loci described above. MNG1 has shown strong evidence of linkage in only one Canadian kindred with multiple multinodular goiters (MNGs) and linkage analyses in 124 additional families failed to find an association between MNG1 and FNMTC. Therefore, the locus may be important for MNG alone but not for FNMTC.[21,22,26,37-39] TCO accounts for a minority of FNMTC cases, but specifically those associated with tumor cell oxyphilia, which is a rare morphology that does not apply to the majority of FNMTC cases ascertained.[22-25] fPTC/PRN is also a rare subtype of FNMTC in which PTC is associated with papillary renal neoplasia, but other than the original family reported, no additional families sharing this phenotype have been identified.[22,26,39] NMTC1 seems to predispose to the follicular variant of PTC, another rare subtype. Classic PTC and oxyphilic tumors are also associated with this locus, though to a lesser extent.[24,24,27] In 2001, a comprehensive mutation and linkage analysis of 22 international FNMTC families revealed that only one family had significant linkage to any known susceptibility locus (TCO in this case), including the ones described above.[22] This cumulative evidence suggests that these FNMTC loci account for disease in a small subset of families, which is consistent with the concept that FNMTC exhibits genetic and locus heterogeneity.
Susceptibility loci identified through genome-wide SNV arrays
Five FNMTC loci have been identified through increasingly dense SNV arrays, also listed in Table 8. The first FNMTC study done by SNV array along with microsatellite analysis was in 2008 in a Portuguese family.[28] This family had five members with PTC (4 classic and 1 follicular variant) and 11 members with benign thyroid diseases. The susceptibility locus was identified at 8p23.1-p22 and designated FTEN (familial thyroid epithelial neoplasia). The 8q24 locus was first identified from a linkage analysis study using SNV arrays of 26 FNMTC families (with PTC). One family had three generations of PTC and melanoma (and MNG); but melanoma was not reported in the other 25 families. Sequencing of genes in the 8q24 region did not reveal any candidate pathogenic variants, but gene expression analysis indicated AK023948 (PTSCC1), a noncoding RNA gene that is downregulated in PTC, could be involved.[29]
In 2009, a population-level study was done in Iceland on 197 cases of PTC or FTC and compared with genotypes of 37,196 Icelandic controls.[30] Two loci had high statistical significance, 9q22.33 and 14q13.3, which are near the genes FOXE1 and NKX2-1, respectively. Two SNVs in particular were associated with increased risk of PTC and FTC: rs944289 (near NKX2-1) and rs965513 (near FOXE1). These results were replicated in two additional large cohorts from the United States (726 individuals tested) and Spain (1,433 individuals tested), as well as other cohorts that also found additional SNVs of interest, particularly in FOXE1.[30,40,41] FOXE1 remains a gene of interest in FNMTC because it produces a thyroid transcription factor with a key role in thyroid gland formation, differentiation, and function.[42] The NKX2.1/TITF-1 gene also encodes a thyroid transcription factor. A germline variant, A339V, has been reported in two FNMTC families affected with MNG or PTC/MNG;[43] however, this association could not be replicated in subsequent studies of other families.[44] Lastly, a large United States and Italian cohort (110 individuals, 49 affected, from 28 FNMTC families) was studied using a 50K SNV array. The majority of these families had classic PTC. The pooled analysis showed linkage to previously identified 1q21 locus (PRN) and a new locus at 6q22.[31]
MicroRNA (miRNA) susceptibility loci
miRNAs are small noncoding RNAs that regulate gene expression. Whole-genome miRNA microarrays were used to evaluate 21 sporadic and seven familial NMTC cases, as well as ten normal thyroid tissue samples.[32] Two miRNAs, miR-20a (13q31.3) and miR-886-3p (5q31.2), were differentially expressed between sporadic and familial NMTC, as confirmed by quantitative reverse transcription-polymerase chain reaction (RT-PCR). Both were also downregulated in NMTC compared with normal thyroid tissues by fourfold. Cell-line transfection studies using miR-886-3p confirmed that it plays a critical role in cell proliferation and migration and it regulates genes involved in DNA replication and focal adhesion pathways.[32] Furthermore, a polymorphism in pre-miR-146a (rs2910164) has been shown to affect miRNA expression and was identified in a significant proportion of the tumors of 608 PTC patients, suggesting it could contribute to genetic predisposition to PTC and play a role in the tumorigenesis through somatic changes.[45] The role of gene regulatory mechanisms and their effect on gene expression and FNMTC tumorigenesis warrants further exploration.
Telomere-telomerase complex
Telomeres are noncoding chromosomal ends consisting of tandem repeats that are important in maintaining chromosomal stability. Telomere length is maintained by a telomerase complex that includes telomerase reverse transcriptase (TERT), along with six other proteins: TRF1, TFR2, RAP1, TIN2, TPP1, and POT1.[46] Shortened telomere length is associated with chromosomal instability that can play a role in cancer development. The telomere-telomerase complex has become a focus of investigation as another possible genetic mechanism for predisposition to FNMTC. In 2008, a cohort of patients with FNMTC was studied using qualitative PCR and fluorescence in situ hybridization to evaluate relative telomere length.[33] They found that telomere length was significantly shorter in familial PTC patients compared with unaffected family members and sporadic PTC. The same group also found that the telomeres in FNMTC cancers were relatively fragile and had a high rate of fragment formation.[47] A second study of telomere length in FNMTC also showed shorter telomere lengths in 13 affected patients compared with 31 unaffected family members.[48] However, the same study showed that relative telomere length was not associated with altered copy number or expression of telomere complex genes hTERT, TRF1, TFR2, RAP1, TIN2, TPP1, or POT1. Other studies have failed to show any significant differences in telomere length between FNMTC and sporadic PTC cases.[49] The role and mechanism of the telomere-telomerase complex in predisposition to FNMTC remains to be elucidated.
Other recently identified FNMTC susceptibility genes and variants
SRGAP1 is a gene that was identified in 2013 through genome-wide linkage analysis of 38 FNMTC families with PTC from the United States and Poland.[34] Four germline missense variants were identified but two variants, Q149H and A275T, were most notable because they segregated in two separate families but not in 800 sporadic cases. SRGAP1 regulates the small G-protein CDC42 in neurons and affects cell mobility.[50] Functional assays demonstrated that Q149H and R617C variants in SRGAP1 could lead to loss-of-function changes that impair ability to inactivate CDC42, which could lead to tumorigenesis.[34] Further studies are needed to validate the association of this gene in other FNMTC cohorts.
HAPB2 was identified in 2015 through whole-exome sequencing (WES) in seven affected members of an FNMTC kindred with PTC and follicular adenoma, using unaffected spouses as controls.[35] One specific germline variant, G534E, was found in the heterozygous state in all affected cases. The group also detected this variant through next-generation sequencing in 4.7% of NMTC cases from the Cancer Genome Atlas. This variant was associated with increased HAPB2 protein expression in the thyroid neoplasms of affected family members. This was not seen in normal thyroid tissue or in individuals with sporadic PTC. Functional G534E studies showed that this variant increased colony formation and cellular migration, suggesting a loss of tumor suppression function. Notably, the authors used a criterion of general population frequency of 1% or less to filter variants identified in this kindred using the 1000 Genomes Project (phase III) and HapMap3. However, subsequent correspondences commented on higher reported frequencies of G534E variants from public databases including that of the Exome Aggregation Consortium (ExAC) (2.22% in the total population, 3.29% in non-Finnish European individuals) and the National Heart, Lung, and Blood Institute (NHLBI) Grand Opportunity Exome Sequencing Project database (5.5% in the total population, 3.88% in American individuals of European descent).[51-53] Many additional studies were conducted to ascertain the frequency of HAPB2 G534E in FNMTC with variable results. While the G534E variant was not identified in 12 Chinese FNMTC families with PTC (nor in 217 patients with sporadic PTC) [53] or in 11 Middle Eastern FNMTC family members,[54] it was shown to segregate in several independent FNMTC kindreds with PTC from a United States study.[55] Several other studies showed that the G534E variant was found in controls and sporadic cases just as often, or more often, than it was found in familial cases of PTC (if the variant was identified in familial PTC cohorts at all). In some cases, the G534E variant did not segregate with disease in familial PTC cohorts.[54,56-58] Therefore, it seems that the HAPB2 G534E variant frequency differs among ancestries and populations; this variant was present in low-to-moderate levels in individuals of European ancestry and in low levels, or completely absent, in individuals of Asian and Middle Eastern ancestries. Larger validation studies are required to determine its role and association with FNMTC.
Lastly, RTFC (c14orf93) was identified through WES of FNMTC families in China.[36] Three genes were identified as candidate genes for FNMTC (RTFC, PYGL, and BMP4) but the RTFC gene was the only one shown to have oncogenic function in promoting thyroid cancer cell survival under starving conditions and promoting cell migration and colony-forming capacity. Specifically, the V205M (c.613G>C) variant in RTFC was important because it was identified in FNMTC patients but absent in unaffected controls. Two additional oncogenic RTFC variants were identified (R115Q and G209D) in patients with sporadic NMTC. Collectively, the ExAC frequencies of these variants in East Asian populations are higher than the ExAC frequencies in the total population, which may indicate that this gene is most relevant in East Asian families with FNMTC. Larger validation studies of this gene and these variants need to be conducted.
In summary, although multiple susceptibility loci have been identified in FNMTC families, no single locus accounts for the majority of nonsyndromic FNMTC and no gene identified shows strong enough associations to warrant clinical genetic testing. Newer sequencing techniques, including WES, will allow for new genes to be discovered and evaluated. Identifying susceptibility genes will allow for screening and early diagnosis, which in turn would lead to improved outcomes for patients and families.
Surveillance
Differentiated thyroid cancer, whether inherited or sporadic, may be associated with a high rate of recurrence, depending on the clinicopathologic features of the disease. Disease recurrence may occur as late as 40 years after initial diagnosis.[59] Surveillance for recurrent disease therefore plays an important role in the long-term management of patients with these tumors. The optimal follow-up strategy is dependent upon both the initial tumor characteristics and the patient's response to therapy.[60] Fortunately, for most patients, the disease is associated with a low risk of recurrence, and surveillance is accordingly less intensive. In these cases, postoperative evaluation is centered on sonographic examination of the neck and measurement of serum thyroglobulin.[60]
Thyroglobulin, a protein produced by both benign and malignant thyroid follicular cells, is used as a tumor marker for patients with differentiated thyroid cancer. Thyroglobulin measurement is most sensitive after a total thyroidectomy, so detection of thyroglobulin—particularly an increasing trend in the serum concentration—is often an early indicator of recurrent or progressive disease.[61] However, it is important to recognize several caveats about the use of this tumor marker. It is imperative to assess serum thyroid-stimulating hormone (TSH) and thyroglobulin antibody levels concomitantly at each measurement. Thyroglobulin rises with increasing TSH values; therefore, an elevating thyroglobulin level could indicate progressive disease or simply a rising TSH level. Furthermore, the presence of thyroglobulin antibodies can interfere with the accurate measurement of thyroglobulin, with most cases resulting in a spurious lowering of the tumor marker.[62] In such cases, the antibody titer may be used as a surrogate marker of disease status.[60] The final caveat about the use of thyroglobulin as a tumor marker is that the test must be performed in the same laboratory at each measurement to accurately assess the trend in levels; each assay can render a different value of thyroglobulin on the same serum sample.[60] Measurement of serum thyroglobulin to assess for recurrent or persistent disease may be performed 3 to 6 months after therapy is completed and monitored periodically thereafter, depending on the concern for persistent or recurrent disease.[60] Stimulated thyroglobulin testing (after withdrawal of thyroid hormone reaches a minimum TSH level of 30 mIU/L or after recombinant TSH injection) may be useful in select patients, particularly in patients with follicular thyroid cancer or in whom there is high clinical suspicion of recurrent or residual disease.
Whether a patient has received radioactive iodine or only surgery, careful ultrasonography of all compartments in the anterior neck is an important tool to determine if there is recurrent or residual disease because most disease is localized in this region. The initial ultrasonography is typically performed 6 to 12 months after surgery.[60] Ultrasonography may be performed sooner if there is concern about residual disease, but it is important for the sonographer to recognize the potential for false-positive findings due to postoperative swelling. The timing and need for subsequent sonographic evaluation of the neck is dependent upon the patient’s risk for recurrence and the serum thyroglobulin status.[60]
Ultrasonography combined with a serum thyroglobulin test has a very high sensitivity for identifying nodal disease, far superior to the radioiodine diagnostic whole-body scans that were historically the mainstay of surveillance.[61]
Interventions
Once a thyroid nodule is detected, further work-up includes complete ultrasonography of the thyroid, as well as a comprehensive neck ultrasonography to evaluate the central and lateral neck lymph nodes. Comprehensive preoperative neck ultrasonography not only provides the opportunity for fine-needle aspiration (FNA) biopsy of any suspicious nodes before surgery but also allows the surgeon to plan the appropriate surgery and counsel the patient regarding a surgical procedure and its associated risks.[63]
FNA is indicated for cytologic evaluation of suspicious nodules based on size of the nodule, imaging characteristics, and associated patient risk factors.[64,65] Most current guidelines recommend consideration of FNA biopsy of all nodules measuring 10 mm or larger. Those nodules with less suspicious sonographic features may be considered for FNA at a larger size threshold. Nodules smaller than 10 mm in greatest dimension may still warrant cytologic evaluation if radiographic imaging demonstrates features concerning for malignancy, such as suspicious lymphadenopathy or extrathyroidal extension. Consideration may also be given to perform FNA of sonographically suspicious nodules, regardless of size, for patients with a strong family history of thyroid cancer. Ultrasonographic features suspicious for malignancy include hypoechogenicity, solid composition, taller-than-wide shape, infiltrative borders, and microcalcifications.
Although positron emission tomography scanning is not recommended for thyroid nodule assessment, concentrated uptake of contrast in the thyroid gland may be detected when the scan is obtained for other reasons. Incidental increase in fluorine F 18-fludeoxyglucose avidity, and an increase in nodule size (more than 50% volume) during surveillance may also be indications for FNA biopsy of nodules.[60]
Cytologic evaluation and indeterminate thyroid nodules
The Bethesda Thyroid Cytology Classification standardizes the cytologic interpretation of thyroid biopsies. Pathologic results are classified into one of the following six categories:[66]
- Nondiagnostic or unsatisfactory.
- Benign.
- Atypia of undetermined significance (AUS) or follicular lesion of undetermined significance (FLUS).
- Follicular neoplasm or suspicious for follicular neoplasm.
- Suspicious for malignancy.
- Malignant.
Patients with biopsy-proven malignant nodules (or nodules suspicious for malignancy) may be considered for surgical resection as discussed below. Nodules classified as AUS/FLUS fall into the indeterminate category because the extent of architectural or cytologic atypia excludes a benign diagnosis, but the degree of atypia is insufficient for a definitive malignant classification.[66] These lesions may be considered for repeat FNA, surveillance ultrasonography, molecular testing, or surgical removal on the basis of clinical concern for malignancy, comorbid conditions, and/or family history of thyroid cancer.[60]
Surgical treatment of thyroid cancer
Patients with a diagnosis of FNMTC may have increased aggressiveness of disease in comparison with sporadic cases.[67] If there are three affected members of a kindred, the tumor is more likely to have aggressive features at a younger age; this suggests a more aggressive surgical management may be warranted.[68] Most experts support total thyroidectomy because of the risk of increased frequency of multicentric disease, lymph node metastases, local invasion, and recurrence of aggressive disease.[67,69] Most surgeons would agree that patients with FNMTC and radiographically, clinically, or intraoperatively suspicious or biopsy-proven metastatic lymph nodes warrant total thyroidectomy and therapeutic compartment-based removal of the lymph node basin(s). Controversy exists, however, as to the appropriate treatment of nonenlarged lymph nodes of the central neck at the time of initial thyroidectomy. Specifically, some groups advocate routine prophylactic central node dissection (PCND) for all patients with known FNMTC to decrease the risk of local recurrence, although there are no specific, prospective, randomized data to support a survival benefit.[70,71] While two retrospective studies (not specific to hereditary thyroid cancer) have reported a reduction in disease recurrence rates associated with PCND,[72,73] two meta-analyses have shown that PCND does not reduce recurrence rates in a clinically significant manner.[74,75] The current recommendations published by the American Thyroid Association (ATA) state that prophylactic or bilateral Level VI lymph node dissection is recommended in patients with T3/T4 papillary cancer (whether familial or not), clinically involved lateral neck nodes or if the information will be used to plan further therapy such as radioactive iodine ablation. The ATA also states that this recommendation should be interpreted in light of available surgical expertise, acknowledging that PCND may lead to increased perioperative morbidity.[60] Currently, selective, rather than routine PCND seems the most reasonable option to guide the decision process.[76]
After total thyroidectomy, patients will need lifelong thyroid hormone replacement therapy.[60] The levothyroxine replacement therapy dose is approximately 1.6 µg/kg/day and is then titrated to reach an appropriate level of TSH suppression.[77,78] The degree of TSH suppression is also individualized on the basis of the patient’s disease status, risk of recurrence, an individual's risk of cardiovascular and bone complications with aggressive TSH suppression,[60] and clinicopathological tumor features. Patients typically undergo a surveillance regimen for recurrence consisting of laboratory evaluation and ultrasonography. In papillary and follicular cancer, thyroxine and TSH demonstrate the level of thyroid suppression, and thyroglobulin and thyroglobulin antibody levels are important markers for possible disease recurrence or metastases.
References
- Davies L, Welch HG: Current thyroid cancer trends in the United States. JAMA Otolaryngol Head Neck Surg 140 (4): 317-22, 2014. [PUBMED Abstract]
- Bhatti P, Veiga LH, Ronckers CM, et al.: Risk of second primary thyroid cancer after radiotherapy for a childhood cancer in a large cohort study: an update from the childhood cancer survivor study. Radiat Res 174 (6): 741-52, 2010. [PUBMED Abstract]
- Mazonakis M, Tzedakis A, Damilakis J, et al.: Thyroid dose from common head and neck CT examinations in children: is there an excess risk for thyroid cancer induction? Eur Radiol 17 (5): 1352-7, 2007. [PUBMED Abstract]
- Pazaitou-Panayiotou K, Capezzone M, Pacini F: Clinical features and therapeutic implication of papillary thyroid microcarcinoma. Thyroid 17 (11): 1085-92, 2007. [PUBMED Abstract]
- Stoffer SS, Van Dyke DL, Bach JV, et al.: Familial papillary carcinoma of the thyroid. Am J Med Genet 25 (4): 775-82, 1986. [PUBMED Abstract]
- Goldgar DE, Easton DF, Cannon-Albright LA, et al.: Systematic population-based assessment of cancer risk in first-degree relatives of cancer probands. J Natl Cancer Inst 86 (21): 1600-8, 1994. [PUBMED Abstract]
- Frich L, Glattre E, Akslen LA: Familial occurrence of nonmedullary thyroid cancer: a population-based study of 5673 first-degree relatives of thyroid cancer patients from Norway. Cancer Epidemiol Biomarkers Prev 10 (2): 113-7, 2001. [PUBMED Abstract]
- Hemminki K, Eng C, Chen B: Familial risks for nonmedullary thyroid cancer. J Clin Endocrinol Metab 90 (10): 5747-53, 2005. [PUBMED Abstract]
- Loh KC: Familial nonmedullary thyroid carcinoma: a meta-review of case series. Thyroid 7 (1): 107-13, 1997. [PUBMED Abstract]
- Lupoli G, Vitale G, Caraglia M, et al.: Familial papillary thyroid microcarcinoma: a new clinical entity. Lancet 353 (9153): 637-9, 1999. [PUBMED Abstract]
- Charkes ND: On the prevalence of familial nonmedullary thyroid cancer in multiply affected kindreds. Thyroid 16 (2): 181-6, 2006. [PUBMED Abstract]
- Lote K, Andersen K, Nordal E, et al.: Familial occurrence of papillary thyroid carcinoma. Cancer 46 (5): 1291-7, 1980. [PUBMED Abstract]
- Houlston RS, Stratton MR: Genetics of non-medullary thyroid cancer. QJM 88 (10): 685-93, 1995. [PUBMED Abstract]
- Burgess JR, Duffield A, Wilkinson SJ, et al.: Two families with an autosomal dominant inheritance pattern for papillary carcinoma of the thyroid. J Clin Endocrinol Metab 82 (2): 345-8, 1997. [PUBMED Abstract]
- Malchoff CD, Malchoff DM: Familial nonmedullary thyroid carcinoma. Cancer Control 13 (2): 106-10, 2006. [PUBMED Abstract]
- Khan A, Smellie J, Nutting C, et al.: Familial nonmedullary thyroid cancer: a review of the genetics. Thyroid 20 (7): 795-801, 2010. [PUBMED Abstract]
- Vriens MR, Suh I, Moses W, et al.: Clinical features and genetic predisposition to hereditary nonmedullary thyroid cancer. Thyroid 19 (12): 1343-9, 2009. [PUBMED Abstract]
- Nosé V: Familial thyroid cancer: a review. Mod Pathol 24 (Suppl 2): S19-33, 2011. [PUBMED Abstract]
- Sturgeon C, Clark OH: Familial nonmedullary thyroid cancer. Thyroid 15 (6): 588-93, 2005. [PUBMED Abstract]
- Hou P, Xing M: Absence of germline mutations in genes within the MAP kinase pathway in familial non-medullary thyroid cancer. Cell Cycle 5 (17): 2036-9, 2006. [PUBMED Abstract]
- Bignell GR, Canzian F, Shayeghi M, et al.: Familial nontoxic multinodular thyroid goiter locus maps to chromosome 14q but does not account for familial nonmedullary thyroid cancer. Am J Hum Genet 61 (5): 1123-30, 1997. [PUBMED Abstract]
- Bevan S, Pal T, Greenberg CR, et al.: A comprehensive analysis of MNG1, TCO1, fPTC, PTEN, TSHR, and TRKA in familial nonmedullary thyroid cancer: confirmation of linkage to TCO1. J Clin Endocrinol Metab 86 (8): 3701-4, 2001. [PUBMED Abstract]
- Canzian F, Amati P, Harach HR, et al.: A gene predisposing to familial thyroid tumors with cell oxyphilia maps to chromosome 19p13.2. Am J Hum Genet 63 (6): 1743-8, 1998. [PUBMED Abstract]
- McKay JD, Thompson D, Lesueur F, et al.: Evidence for interaction between the TCO and NMTC1 loci in familial non-medullary thyroid cancer. J Med Genet 41 (6): 407-12, 2004. [PUBMED Abstract]
- Prazeres HJ, Rodrigues F, Soares P, et al.: Loss of heterozygosity at 19p13.2 and 2q21 in tumours from familial clusters of non-medullary thyroid carcinoma. Fam Cancer 7 (2): 141-9, 2008. [PUBMED Abstract]
- Malchoff CD, Sarfarazi M, Tendler B, et al.: Papillary thyroid carcinoma associated with papillary renal neoplasia: genetic linkage analysis of a distinct heritable tumor syndrome. J Clin Endocrinol Metab 85 (5): 1758-64, 2000. [PUBMED Abstract]
- McKay JD, Lesueur F, Jonard L, et al.: Localization of a susceptibility gene for familial nonmedullary thyroid carcinoma to chromosome 2q21. Am J Hum Genet 69 (2): 440-6, 2001. [PUBMED Abstract]
- Cavaco BM, Batista PF, Sobrinho LG, et al.: Mapping a new familial thyroid epithelial neoplasia susceptibility locus to chromosome 8p23.1-p22 by high-density single-nucleotide polymorphism genome-wide linkage analysis. J Clin Endocrinol Metab 93 (11): 4426-30, 2008. [PUBMED Abstract]
- He H, Nagy R, Liyanarachchi S, et al.: A susceptibility locus for papillary thyroid carcinoma on chromosome 8q24. Cancer Res 69 (2): 625-31, 2009. [PUBMED Abstract]
- Gudmundsson J, Sulem P, Gudbjartsson DF, et al.: Common variants on 9q22.33 and 14q13.3 predispose to thyroid cancer in European populations. Nat Genet 41 (4): 460-4, 2009. [PUBMED Abstract]
- Suh I, Filetti S, Vriens MR, et al.: Distinct loci on chromosome 1q21 and 6q22 predispose to familial nonmedullary thyroid cancer: a SNP array-based linkage analysis of 38 families. Surgery 146 (6): 1073-80, 2009. [PUBMED Abstract]
- Xiong Y, Zhang L, Holloway AK, et al.: MiR-886-3p regulates cell proliferation and migration, and is dysregulated in familial non-medullary thyroid cancer. PLoS One 6 (10): e24717, 2011. [PUBMED Abstract]
- Capezzone M, Cantara S, Marchisotta S, et al.: Short telomeres, telomerase reverse transcriptase gene amplification, and increased telomerase activity in the blood of familial papillary thyroid cancer patients. J Clin Endocrinol Metab 93 (10): 3950-7, 2008. [PUBMED Abstract]
- He H, Bronisz A, Liyanarachchi S, et al.: SRGAP1 is a candidate gene for papillary thyroid carcinoma susceptibility. J Clin Endocrinol Metab 98 (5): E973-80, 2013. [PUBMED Abstract]
- Gara SK, Jia L, Merino MJ, et al.: Germline HABP2 Mutation Causing Familial Nonmedullary Thyroid Cancer. N Engl J Med 373 (5): 448-55, 2015. [PUBMED Abstract]
- Liu C, Yu Y, Yin G, et al.: C14orf93 (RTFC) is identified as a novel susceptibility gene for familial nonmedullary thyroid cancer. Biochem Biophys Res Commun 482 (4): 590-596, 2017. [PUBMED Abstract]
- McKay JD, Williamson J, Lesueur F, et al.: At least three genes account for familial papillary thyroid carcinoma: TCO and MNG1 excluded as susceptibility loci from a large Tasmanian family. Eur J Endocrinol 141 (2): 122-5, 1999. [PUBMED Abstract]
- Lesueur F, Stark M, Tocco T, et al.: Genetic heterogeneity in familial nonmedullary thyroid carcinoma: exclusion of linkage to RET, MNG1, and TCO in 56 families. NMTC Consortium. J Clin Endocrinol Metab 84 (6): 2157-62, 1999. [PUBMED Abstract]
- Cavaco BM, Batista PF, Martins C, et al.: Familial non-medullary thyroid carcinoma (FNMTC): analysis of fPTC/PRN, NMTC1, MNG1 and TCO susceptibility loci and identification of somatic BRAF and RAS mutations. Endocr Relat Cancer 15 (1): 207-15, 2008. [PUBMED Abstract]
- Bullock M, Duncan EL, O'Neill C, et al.: Association of FOXE1 polyalanine repeat region with papillary thyroid cancer. J Clin Endocrinol Metab 97 (9): E1814-9, 2012. [PUBMED Abstract]
- Landa I, Ruiz-Llorente S, Montero-Conde C, et al.: The variant rs1867277 in FOXE1 gene confers thyroid cancer susceptibility through the recruitment of USF1/USF2 transcription factors. PLoS Genet 5 (9): e1000637, 2009. [PUBMED Abstract]
- Pereira JS, da Silva JG, Tomaz RA, et al.: Identification of a novel germline FOXE1 variant in patients with familial non-medullary thyroid carcinoma (FNMTC). Endocrine 49 (1): 204-14, 2015. [PUBMED Abstract]
- Ngan ES, Lang BH, Liu T, et al.: A germline mutation (A339V) in thyroid transcription factor-1 (TITF-1/NKX2.1) in patients with multinodular goiter and papillary thyroid carcinoma. J Natl Cancer Inst 101 (3): 162-75, 2009. [PUBMED Abstract]
- Cantara S, Capuano S, Formichi C, et al.: Lack of germline A339V mutation in thyroid transcription factor-1 (TITF-1/NKX2.1) gene in familial papillary thyroid cancer. Thyroid Res 3 (1): 4, 2010. [PUBMED Abstract]
- Jazdzewski K, Murray EL, Franssila K, et al.: Common SNP in pre-miR-146a decreases mature miR expression and predisposes to papillary thyroid carcinoma. Proc Natl Acad Sci U S A 105 (20): 7269-74, 2008. [PUBMED Abstract]
- Fu D, Collins K: Purification of human telomerase complexes identifies factors involved in telomerase biogenesis and telomere length regulation. Mol Cell 28 (5): 773-85, 2007. [PUBMED Abstract]
- Cantara S, Pisu M, Frau DV, et al.: Telomere abnormalities and chromosome fragility in patients affected by familial papillary thyroid cancer. J Clin Endocrinol Metab 97 (7): E1327-31, 2012. [PUBMED Abstract]
- He M, Bian B, Gesuwan K, et al.: Telomere length is shorter in affected members of families with familial nonmedullary thyroid cancer. Thyroid 23 (3): 301-7, 2013. [PUBMED Abstract]
- Jendrzejewski J, Tomsic J, Lozanski G, et al.: Telomere length and telomerase reverse transcriptase gene copy number in patients with papillary thyroid carcinoma. J Clin Endocrinol Metab 96 (11): E1876-80, 2011. [PUBMED Abstract]
- Wong K, Ren XR, Huang YZ, et al.: Signal transduction in neuronal migration: roles of GTPase activating proteins and the small GTPase Cdc42 in the Slit-Robo pathway. Cell 107 (2): 209-21, 2001. [PUBMED Abstract]
- Tomsic J, He H, de la Chapelle A: HABP2 Mutation and Nonmedullary Thyroid Cancer. N Engl J Med 373 (21): 2086, 2015. [PUBMED Abstract]
- Sponziello M, Durante C, Filetti S: HABP2 Mutation and Nonmedullary Thyroid Cancer. N Engl J Med 373 (21): 2085-6, 2015. [PUBMED Abstract]
- Zhou EY, Lin Z, Yang Y: HABP2 Mutation and Nonmedullary Thyroid Cancer. N Engl J Med 373 (21): 2084-5, 2015. [PUBMED Abstract]
- Alzahrani AS, Murugan AK, Qasem E, et al.: HABP2 Gene Mutations Do Not Cause Familial or Sporadic Non-Medullary Thyroid Cancer in a Highly Inbred Middle Eastern Population. Thyroid 26 (5): 667-71, 2016. [PUBMED Abstract]
- Zhang T, Xing M: HABP2 G534E Mutation in Familial Nonmedullary Thyroid Cancer. J Natl Cancer Inst 108 (6): djv415, 2016. [PUBMED Abstract]
- Tomsic J, Fultz R, Liyanarachchi S, et al.: HABP2 G534E Variant in Papillary Thyroid Carcinoma. PLoS One 11 (1): e0146315, 2016. [PUBMED Abstract]
- Sahasrabudhe R, Stultz J, Williamson J, et al.: The HABP2 G534E variant is an unlikely cause of familial non-medullary thyroid cancer. J Clin Endocrinol Metab 10 (3): 1098-1103, 2016. [PUBMED Abstract]
- Weeks AL, Wilson SG, Ward L, et al.: HABP2 germline variants are uncommon in familial nonmedullary thyroid cancer. BMC Med Genet 17 (1): 60, 2016. [PUBMED Abstract]
- Mazzaferri EL, Jhiang SM: Long-term impact of initial surgical and medical therapy on papillary and follicular thyroid cancer. Am J Med 97 (5): 418-28, 1994. [PUBMED Abstract]
- Haugen BR, Alexander EK, Bible KC, et al.: 2015 American Thyroid Association Management Guidelines for Adult Patients with Thyroid Nodules and Differentiated Thyroid Cancer: The American Thyroid Association Guidelines Task Force on Thyroid Nodules and Differentiated Thyroid Cancer. Thyroid 26 (1): 1-133, 2016. [PUBMED Abstract]
- Pacini F, Molinaro E, Castagna MG, et al.: Recombinant human thyrotropin-stimulated serum thyroglobulin combined with neck ultrasonography has the highest sensitivity in monitoring differentiated thyroid carcinoma. J Clin Endocrinol Metab 88 (8): 3668-73, 2003. [PUBMED Abstract]
- Spencer CA, Takeuchi M, Kazarosyan M, et al.: Serum thyroglobulin autoantibodies: prevalence, influence on serum thyroglobulin measurement, and prognostic significance in patients with differentiated thyroid carcinoma. J Clin Endocrinol Metab 83 (4): 1121-7, 1998. [PUBMED Abstract]
- Marshall CL, Lee JE, Xing Y, et al.: Routine pre-operative ultrasonography for papillary thyroid cancer: effects on cervical recurrence. Surgery 146 (6): 1063-72, 2009. [PUBMED Abstract]
- Pellegriti G, Frasca F, Regalbuto C, et al.: Worldwide increasing incidence of thyroid cancer: update on epidemiology and risk factors. J Cancer Epidemiol 2013: 965212, 2013. [PUBMED Abstract]
- Kwak JY: Indications for fine needle aspiration in thyroid nodules. Endocrinol Metab (Seoul) 28 (2): 81-5, 2013. [PUBMED Abstract]
- Cibas ES, Ali SZ: The Bethesda System for Reporting Thyroid Cytopathology. Thyroid 19 (11): 1159-65, 2009. [PUBMED Abstract]
- Mazeh H, Benavidez J, Poehls JL, et al.: In patients with thyroid cancer of follicular cell origin, a family history of nonmedullary thyroid cancer in one first-degree relative is associated with more aggressive disease. Thyroid 22 (1): 3-8, 2012. [PUBMED Abstract]
- El Lakis M, Giannakou A, Nockel PJ, et al.: Do patients with familial nonmedullary thyroid cancer present with more aggressive disease? Implications for initial surgical treatment. Surgery 165 (1): 50-57, 2019. [PUBMED Abstract]
- Mazeh H, Sippel RS: Familial nonmedullary thyroid carcinoma. Thyroid 23 (9): 1049-56, 2013. [PUBMED Abstract]
- Mazzaferri EL, Doherty GM, Steward DL: The pros and cons of prophylactic central compartment lymph node dissection for papillary thyroid carcinoma. Thyroid 19 (7): 683-9, 2009. [PUBMED Abstract]
- McLeod DS, Sawka AM, Cooper DS: Controversies in primary treatment of low-risk papillary thyroid cancer. Lancet 381 (9871): 1046-57, 2013. [PUBMED Abstract]
- Moo TA, McGill J, Allendorf J, et al.: Impact of prophylactic central neck lymph node dissection on early recurrence in papillary thyroid carcinoma. World J Surg 34 (6): 1187-91, 2010. [PUBMED Abstract]
- Perrino M, Vannucchi G, Vicentini L, et al.: Outcome predictors and impact of central node dissection and radiometabolic treatments in papillary thyroid cancers < or =2 cm. Endocr Relat Cancer 16 (1): 201-10, 2009. [PUBMED Abstract]
- Shan CX, Zhang W, Jiang DZ, et al.: Routine central neck dissection in differentiated thyroid carcinoma: a systematic review and meta-analysis. Laryngoscope 122 (4): 797-804, 2012. [PUBMED Abstract]
- Zetoune T, Keutgen X, Buitrago D, et al.: Prophylactic central neck dissection and local recurrence in papillary thyroid cancer: a meta-analysis. Ann Surg Oncol 17 (12): 3287-93, 2010. [PUBMED Abstract]
- Moreno MA, Edeiken-Monroe BS, Siegel ER, et al.: In papillary thyroid cancer, preoperative central neck ultrasound detects only macroscopic surgical disease, but negative findings predict excellent long-term regional control and survival. Thyroid 22 (4): 347-55, 2012. [PUBMED Abstract]
- Jin J, Allemang MT, McHenry CR: Levothyroxine replacement dosage determination after thyroidectomy. Am J Surg 205 (3): 360-3; discussion 363-4, 2013. [PUBMED Abstract]
- Mistry D, Atkin S, Atkinson H, et al.: Predicting thyroxine requirements following total thyroidectomy. Clin Endocrinol (Oxf) 74 (3): 384-7, 2011. [PUBMED Abstract]
Latest Updates to This Summary (12/13/2024)
The PDQ cancer information summaries are reviewed regularly and updated as new information becomes available. This section describes the latest changes made to this summary as of the date above.
Multiple Endocrine Neoplasia Type 2
This section was extensively revised.
This summary is written and maintained by the PDQ Cancer Genetics Editorial Board, which is editorially independent of NCI. The summary reflects an independent review of the literature and does not represent a policy statement of NCI or NIH. More information about summary policies and the role of the PDQ Editorial Boards in maintaining the PDQ summaries can be found on the About This PDQ Summary and PDQ® Cancer Information for Health Professionals pages.
About This PDQ Summary
Purpose of This Summary
This PDQ cancer information summary for health professionals provides comprehensive, peer-reviewed, evidence-based information about the genetics of endocrine and neuroendocrine neoplasias. It is intended as a resource to inform and assist clinicians in the care of their patients. It does not provide formal guidelines or recommendations for making health care decisions.
Reviewers and Updates
This summary is reviewed regularly and updated as necessary by the PDQ Cancer Genetics Editorial Board, which is editorially independent of the National Cancer Institute (NCI). The summary reflects an independent review of the literature and does not represent a policy statement of NCI or the National Institutes of Health (NIH).
Board members review recently published articles each month to determine whether an article should:
- be discussed at a meeting,
- be cited with text, or
- replace or update an existing article that is already cited.
Changes to the summaries are made through a consensus process in which Board members evaluate the strength of the evidence in the published articles and determine how the article should be included in the summary.
The lead reviewers for Genetics of Endocrine and Neuroendocrine Neoplasias are:
- Erica Blouch, MS, CGC (Massachusetts General Hospital Cancer Center)
- Kathleen A. Calzone, PhD, RN, AGN-BC, FAAN (National Cancer Institute)
- Suzanne C. O'Neill, PhD (Georgetown University)
- Nancy D. Perrier, MD, FACS (University of Texas, M.D. Anderson Cancer Center)
- John M. Quillin, PhD, MPH, MS (Virginia Commonwealth University)
- Charite Ricker, MS, CGC (University of Southern California)
- Catharine Wang, PhD, MSc (Boston University School of Public Health)
Any comments or questions about the summary content should be submitted to Cancer.gov through the NCI website's Email Us. Do not contact the individual Board Members with questions or comments about the summaries. Board members will not respond to individual inquiries.
Levels of Evidence
Some of the reference citations in this summary are accompanied by a level-of-evidence designation. These designations are intended to help readers assess the strength of the evidence supporting the use of specific interventions or approaches. The PDQ Cancer Genetics Editorial Board uses a formal evidence ranking system in developing its level-of-evidence designations.
Permission to Use This Summary
PDQ is a registered trademark. Although the content of PDQ documents can be used freely as text, it cannot be identified as an NCI PDQ cancer information summary unless it is presented in its entirety and is regularly updated. However, an author would be permitted to write a sentence such as “NCI’s PDQ cancer information summary about breast cancer prevention states the risks succinctly: [include excerpt from the summary].”
The preferred citation for this PDQ summary is:
PDQ® Cancer Genetics Editorial Board. PDQ Genetics of Endocrine and Neuroendocrine Neoplasias. Bethesda, MD: National Cancer Institute. Updated <MM/DD/YYYY>. Available at: https://www.cancer.gov/types/thyroid/hp/medullary-thyroid-genetics-pdq. Accessed <MM/DD/YYYY>. [PMID: 26389271]
Images in this summary are used with permission of the author(s), artist, and/or publisher for use within the PDQ summaries only. Permission to use images outside the context of PDQ information must be obtained from the owner(s) and cannot be granted by the National Cancer Institute. Information about using the illustrations in this summary, along with many other cancer-related images, is available in Visuals Online, a collection of over 2,000 scientific images.
Disclaimer
The information in these summaries should not be used as a basis for insurance reimbursement determinations. More information on insurance coverage is available on Cancer.gov on the Managing Cancer Care page.
Contact Us
More information about contacting us or receiving help with the Cancer.gov website can be found on our Contact Us for Help page. Questions can also be submitted to Cancer.gov through the website’s Email Us.